Introduction
Germanium electro-absorption modulators (Ge EAMs) are promising candidates for energy-efficient and high-speed optical transmitters in silicon photonics. Recently, Ge EAMs with 3-dB bandwidths exceeding 110 GHz have been demonstrated, enabling symbol rates of 100-200 GBaud. However, besides high-speed operation, low insertion losses and sufficiently large extinction ratios are crucial performance metrics for EAMs.
This article discusses the differences between static and dynamic extinction ratios of Ge EAMs and their dependency on optical input power. We explore the commonly used figure of merit, the quotient of static extinction ratio and insertion loss, and argue why this metric may be inappropriate for evaluating Ge EAMs without considering optical power.
Static vs Dynamic Extinction Ratio
The static extinction ratio is typically extracted from transmission curve measurements, where the optical transmission through the EAM is measured at different applied voltages. The dynamic extinction ratio, on the other hand, is determined from eye diagrams captured during high-speed operation of the EAM.
Recent literature has revealed mismatches between the static and dynamic extinction ratios of Ge EAMs to varying degrees. One potential root cause for this mismatch is self-heating effects within the EAM.
Experimental Investigation
To investigate the optical power dependency, transmission curves and eye diagrams were measured on >100 GHz Ge EAMs with a 20 μm length and ~500 nm thick Ge layers.
The static extinction ratios were extracted from the transmission curves measured from 1560 nm to 1620 nm at optical input powers of 0 dBm, 4 dBm, and 8 dBm (at the fiber tip) and applied voltages from 0 V to -3 V.
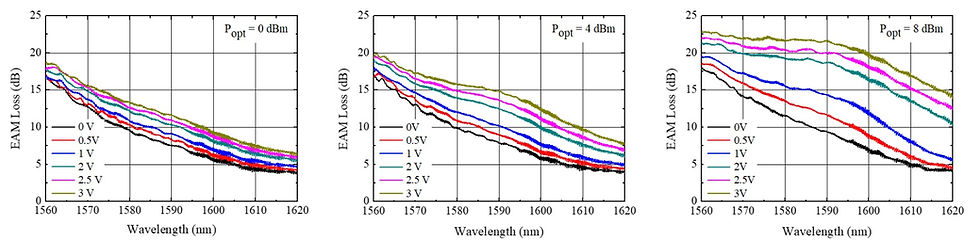
The results in Figure 2 show a substantial increase in static extinction ratio with higher optical input power. At 1600 nm and -3 V bias, the static ER increases from 3.8 dB at 0 dBm to 13 dB at 8 dBm.

For dynamic measurements, eye diagrams were captured at 64 Gbps with the EAM biased at -1.5 V and modulated with a 2 Vpp NRZ signal.
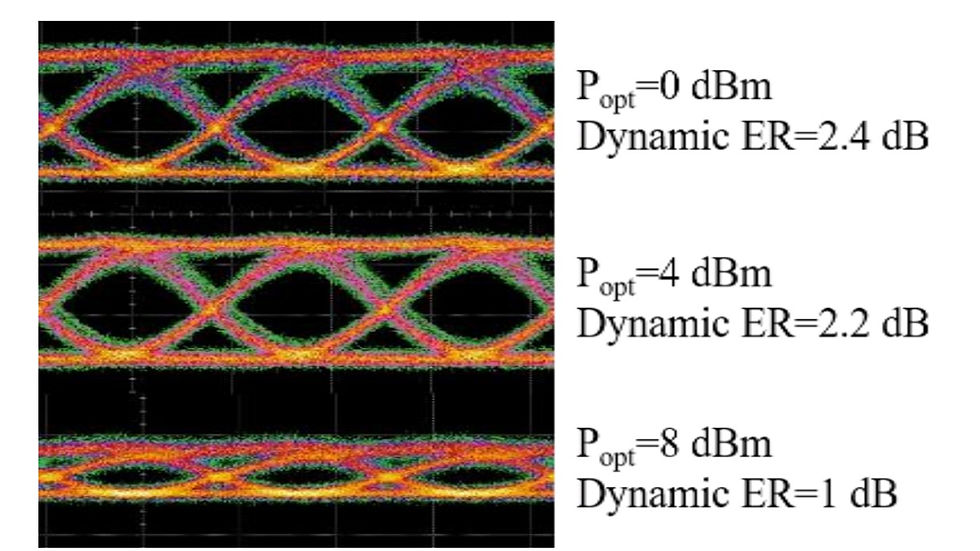
Unlike the static case, the dynamic extinction ratio degrades with increased optical input power, as summarized in Table 1.
Table 1: Dynamic and static ER of EAM and corresponding photocurrents.
Popt | Dynamic ER (2Vpp) | Average Iphoto (dynamic) | Static ER (-2V) | Iphoto (0 V) (static) | Iphoto (-2 V) (static) |
0 dBm | 2.4 dB | 0.3 mA | 2.4 dB | 0.3 mA | 0.4 mA |
4 dBm | 2.25 dB | 0.9 mA | 4 dB | 0.84 mA | 1.1 mA |
8 dBm | 1 dB | 2.8 mA | 9.4 dB | 2.2 mA | 3 mA |
Explanation of Observed Behavior
The authors attribute the opposing trends in static and dynamic extinction ratios to self-heating effects within the Ge EAM. With increasing optical power, the generated photocurrent within the Ge region also increases. This photocurrent induces Joule heating, leading to bandgap shrinkage and further absorption increase at L-band wavelengths via the Franz-Keldysh effect.
The static extinction ratio is partly determined by the difference in photocurrent-induced temperature between the on-state (0 V, 'transparency' mode) and off-state (Vbias, 'absorption' mode). During dynamic operation, however, the temperature at on and off states is governed by the average photocurrent. While the Franz-Keldysh effect can respond at 100 GHz speeds, the thermally induced bandgap change is too slow to follow the applied RF signal. Hence, the maximum dynamic extinction ratio is solely determined by the Franz-Keldysh effect and degrades with increased self-heating at higher optical powers.
Conclusion
This tutorial highlights the diverging behavior of static and dynamic extinction ratios in Ge EAMs and their dependency on optical input power. The commonly used figure of merit based on static extinction ratio and insertion loss may be inappropriate for evaluating Ge EAMs without considering optical power and self-heating effects.
The consideration of static extinction ratio alone may lead to a misleading evaluation of Ge EAM performance, especially when operating at high optical powers that induce substantial self-heating. Both static and dynamic characteristics must be carefully examined to accurately assess the performance of Ge electro-absorption modulators.
Reference
[1] D. Steckler, S. Lischke, A. Peczek, and L. Zimmermann, "On the Dynamic and Static Extinction Ratio of Germanium Electro-Absorption Modulators," IHP, Frankfurt (Oder), Germany; Technische Universität Berlin, Berlin, Germany, 2024.
Comments