Introduction
As traffic toward and inside datacenters is increasing, the power consumption of electrical wiring in ethernet switches needs to be addressed. Co-packaged optics (CPO) is a promising solution, where optical components are integrated into the same package substrate as electrical elements. One of the proposed CPO configurations is the active optical package (AOP) substrate, which uses micro-mirror-based optical redistribution for low-loss and broadband optical coupling between silicon photonics and polymer waveguides.
Considering the practical use of CPO modules in datacenters and network nodes, their operation at high temperatures is necessary. The proposed optical redistribution comprises materials with vastly different coefficients of thermal expansion (CTE), so thermal deformation can affect the coupling efficiency at high temperatures. This study analyzes the thermo-optical characteristics of the mirror-based optical redistribution in the C-band both numerically and experimentally.
Optical Redistribution Design
A. Schematic of Optical Redistribution
Figure 1 shows a schematic of the AOP substrate. It consists of a silicon photonic die embedded in an organic package substrate, with polymer waveguides integrated to link the optical elements on the board. A micro-mirror-based optical redistribution is adopted to couple the silicon photonics and polymer waveguides. It has a silicon waveguide, polymer waveguide, and a pair of 3D micro-mirrors. The top-side mirror has a linear angle, and the bottom-side mirror is curved to convert the spot size.

B. Optimizing Design at Room Temperature
The design parameters of the optical redistribution were optimized at room temperature (20°C) using optical propagation analysis. The key parameters include the curvatures of the bottom-side mirror, distance between the silicon waveguide and bottom-side mirror, and decentering parameters of the mirrors. An inverse-taper spot-size converter was used at the edge of the silicon waveguide. The optimized design achieved a coupling efficiency of 95.7% at 1550 nm.
Thermal Characteristics Analysis
A. Analyzing Thermal Deformation
Thermal deformation of the optical redistribution was calculated using finite element analysis in ANSYS Mechanical. The materials and their thermal properties are shown in Table 1. The maximum total deformation at 85°C was 0.14 μm for the silicon waveguide, 0.25 μm for the bottom-side mirror, 0.87 μm for the polymer waveguide, and 1.16 μm for the top-side mirror (Fig. 2). The polymer cladding and core materials have a larger CTE, causing more deformation in the top-side mirror and polymer waveguide.
Table 1. THERMAL PROPERTY OF MATERIALS COMPOSING OPTICAL REDISTRIBUTION
Material | Young's Modulus [Gpal | Poisson's ratio | Coefficient of thermal expansion [°C-1] |
Polymer Cladding | 0.5 [23] | 0.4 | 1.8x 10-4 [23] |
Polymer Core | 1.0 [23] | 0.4 | 1.5x 10-4 [23] |
Polyimide | 4.0 | 0.34 | 4.0x 10-5 |
Cladding (SiO2) | 73 | 0.155 | 5.55x 10-7 |
Silicon | 169 (x, z) 130 (v) [24] | 0.36 (x-y) 0.28 (y-z) 0.064 (z-x) [24] | 2.59x 10-6 [25] |
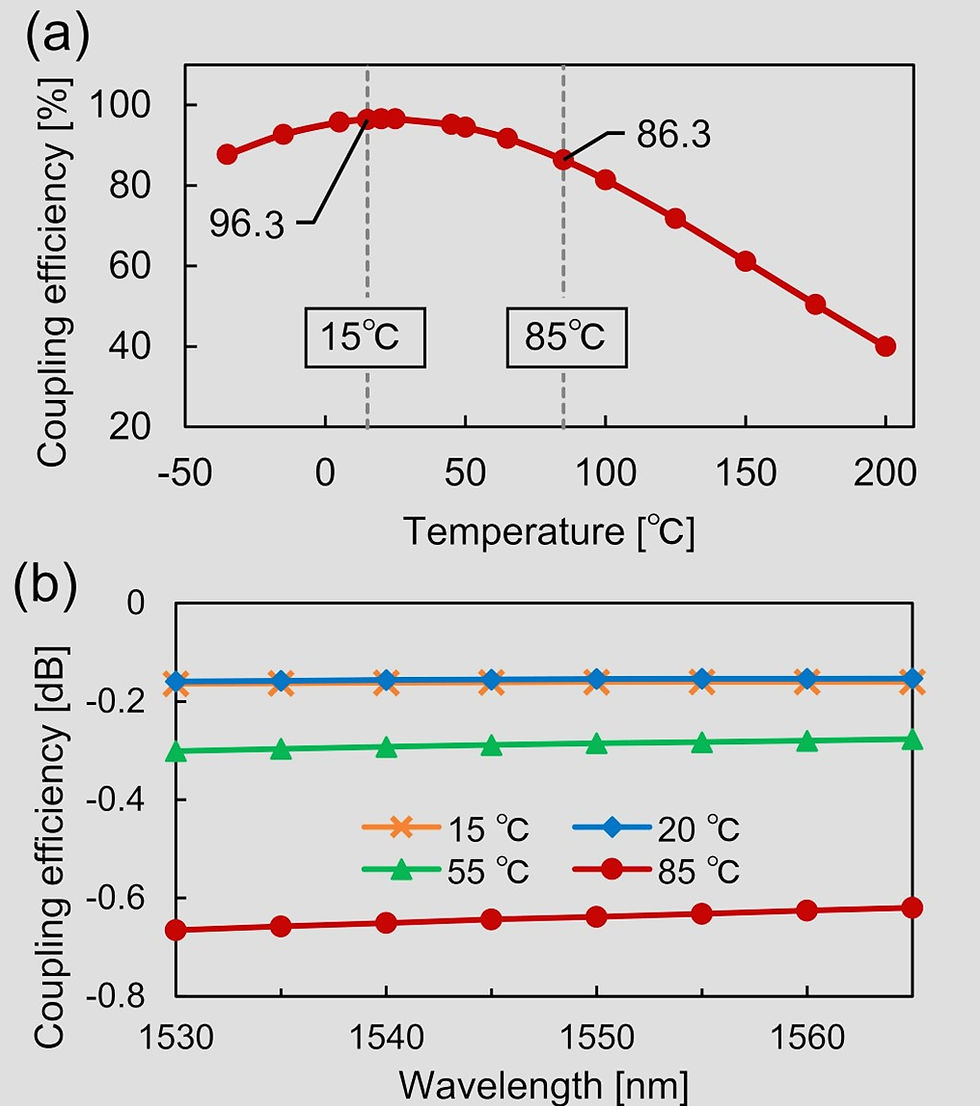
B. Fitting with 2D Polynomial
The deformed mirror surfaces were fitted with a fifth-order 2D polynomial for import into the optical software Zemax OpticStudio. The average root mean squared error was less than 1 nm for the bottom-side mirror and 9 nm for the top-side mirror in the temperature range of -35 to 200°C.
C. Analyzing Temperature-dependent Coupling Efficiency
The thermally deformed mirror surfaces and waveguide positions were used in Zemax to calculate the temperature-dependent coupling efficiency. As shown in Fig. 3(a), more than 85% coupling efficiency was obtained at 1550 nm in the 15-85°C operating temperature range. Figure 3(b) shows the wavelength-dependent coupling efficiency in the C-band, with a difference in average coupling loss between 20°C and 85°C of 0.49 dB.
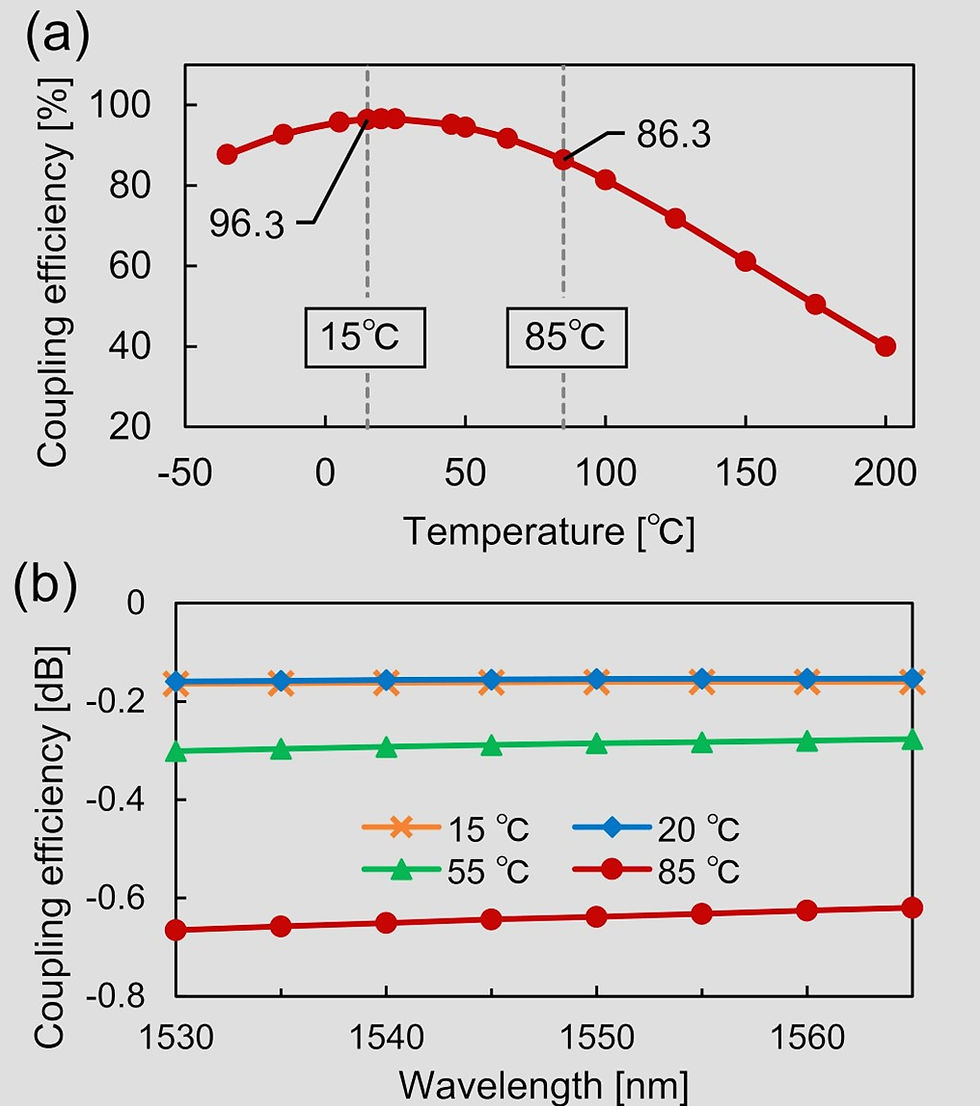
D. Discussion of Calculation Results
Analysis of the loss factors showed that the angle change of the mirrors, especially the top-side mirror made of polymer, had the most significant impact on coupling loss at high temperatures. To address this, the design was adjusted to set the top-side mirror angle to 44.7° at room temperature, reducing the angle mismatch in the 15-85°C range. This modified design achieved a coupling efficiency of more than 92% in the specified temperature range (Fig. 4).
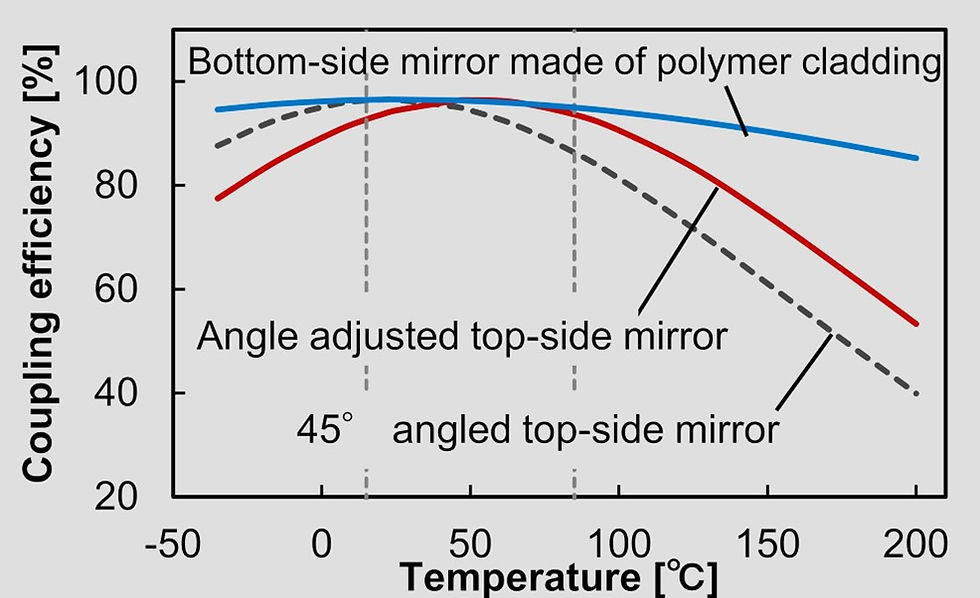
Experimental Demonstration
A device-under-test (DUT) sample with the original room-temperature-optimized optical redistribution design was fabricated and its thermal characteristics were experimentally demonstrated. The setup is shown in Fig. 5.
The transmittance of the optical redistribution in the C-band was measured at 25°C, 55°C, and 85°C. As shown in Fig. 5(d), the average insertion loss difference was 0.80 dB among the three temperatures, confirming the low temperature dependence observed in the analysis.
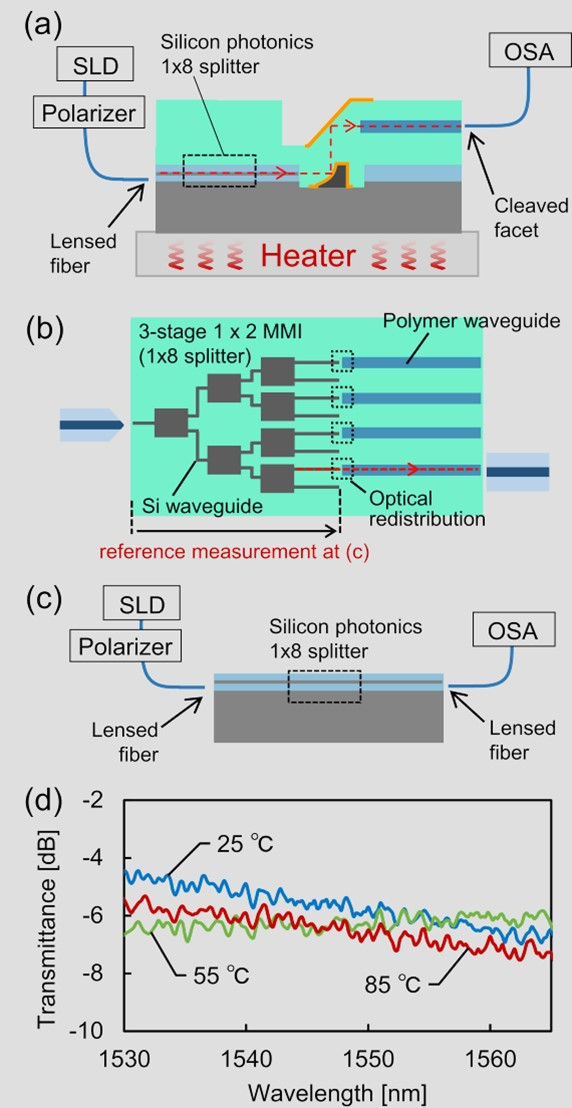
Conclusion
The thermal characteristics of a micro-mirror-based optical redistribution for CPO were analyzed both numerically and experimentally. The calculated coupling efficiency remained above 85% in the 15-85°C temperature range, and the measured transmittance showed an average loss difference of only 0.80 dB across the C-band at different temperatures. By adjusting the top-mirror angle, the coupling efficiency can be maintained above 92% in the specified temperature range. These results demonstrate the thermal stability of the mirror-based optical coupling approach for CPO applications.
Reference
[2] F. Nakamura, S. Suda, T. Kurosu, Y. Ibusuki, A. Noriki, A. Ukita, K. Takemura, T. Aoki, and T. Amano, "Thermal Characteristics of Mirror-based Optical Redistribution for Co-packaged Optics," in IEEE/OSA Journal of Lightwave Technology
Comments