Introduction
Acousto-optic modulators (AOMs) are crucial components that enable the transduction of information between the acoustic and optical domains. They find applications in various fields, including microwave photonics, optical frequency shifting, non-reciprocal photonics, sensing, spectroscopy, and quantum information control. Conventionally, AOMs have been implemented using bulk-crystalline materials, which, despite their excellent performance, are often bulky, expensive, and unsuitable for large-scale integrated applications due to weak acoustic wave confinement.
Surface acoustic wave (SAW) based modulators offer a high degree of acoustic wave confinement on the surface of the structure, allowing them to be co-integrated with photonic devices on the same platform. This tutorial article discusses the realization of a highly efficient, wideband AlScN-Si acousto-optic modulator on a commercially available silicon photonics process, enabling high-yield, low-cost mass production of AOMs integrated with other photonic and electronic devices on the same chip.
Device Structure and Operating Principle
The implemented AOM structure consists of a single-mode optical waveguide fabricated using the AMF silicon-on-insulator (SOI) process and an acoustic transducer fabricated next to the optical waveguide using a CMOS-compatible, in-house post-processing step. The acoustic transducer is realized by depositing an AlScN piezoelectric film on the buried oxide layer (BOX) of the SOI chip, followed by the fabrication of gold interdigitated finger electrodes over the AlScN layer.
When an RF signal is applied to the acoustic transducer, a SAW is launched that propagates on the surface of the BOX and interacts with the optical waveguide. As a result, the optical waveguide experiences strain, causing a change in its refractive index proportional to the acoustic wave strength. This acoustic-induced refractive index change modulates the phase of the optical wave traveling within the waveguide, leading to the appearance of acousto-optic phase modulation sidebands around the optical carrier.
The ratio of the power in the first upper or lower sideband to the power in the carrier is defined as the AOM efficiency (η_AOM), which can be expressed as:
η_AOM = 20 log ((α(L))^2) = P(ω_o ∓ Ω)_dB - P(ω_o)_dB
Where ω_o and Ω are the angular frequencies of the optical wave and the RF signal, respectively, α(L) is the amount of optical phase shift due to acousto-optic modulation, and Δn_eff is the acoustically induced effective index change.
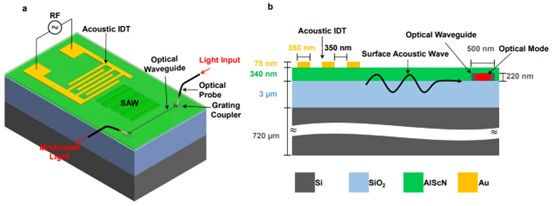
Device Fabrication
The fabrication process involves several steps, as illustrated in Figure 2 of the attached PDF. First, a 340 nm thick layer of AlScN is sputtered on the exposed silicon-on-oxide layer of the photonic chip using a pulsed DC physical vapor deposition (PVD) system. Next, e-beam resist is spin-coated and patterned on the chip to define the interdigitated electrodes. A 75 nm thick layer of gold (with a 10 nm titanium adhesion layer) is then evaporated and patterned using a lift-off process to fabricate the IDT electrodes.
Photoresist is spin-coated and patterned to form low-resistance bus lines and pads for the acoustic transducer. Finally, a 400 nm thick layer of gold (with a 10 nm titanium adhesion layer) is evaporated and patterned using a lift-off process to fabricate the bus lines and pads.
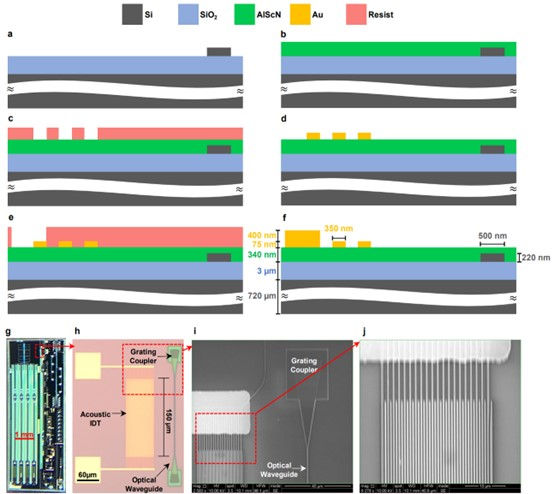
Characterization and Performance
The fabricated acoustic transducer on the commercial photonic chip was characterized using a vector network analyzer (VNA). The electrical reflection coefficient (S11) response of the acoustic transducer as a function of frequency was measured, revealing two strong responses at approximately 4.5 GHz and 5.5 GHz, following the sin(x)/x profile expected for SAW transducers.
The propagation modes for the two transducers were simulated using COMSOL Multiphysics, confirming the presence of two dominant modes at 4.5 GHz and 5.5 GHz. The simulation results showed that while both modes support the propagation of surface acoustic waves, the strain in the silicon waveguide associated with the mode at 5.5 GHz is stronger than that of the mode at 4.5 GHz, indicating a relatively stronger acousto-optic modulation at 5.5 GHz.
The modulation efficiency of the implemented AOM device was measured using a heterodyne detection scheme, as depicted in Figure 3. The output of a tunable laser (Agilent 8164A) emitting at 1550 nm is coupled into the chip using a grating coupler, and the modulated light is coupled out, combined with the output of another tunable laser, and photo-detected. The frequency of the RF synthesizer is swept from 2 GHz to 6 GHz to find the frequency response of the AOM.
The results, shown in Figure 4, demonstrate that the device with 20 IDT finger pairs achieves a modulation efficiency of -18.3 dB measured at 23.2 dBm RF input power, corresponding to a Vπ·L of 1.12 V-cm. Furthermore, the device exhibits a modulation bandwidth of 112 MHz (around 5.5 GHz), resulting in about an order-of-magnitude BW/(Vπ·L) figure-of-merit improvement over state-of-the-art reported silicon-based CMOS-compatible AOMs.
The effect of the number of IDT finger pairs on the device performance was also studied. A device with 5 finger pairs achieved a peak modulation efficiency of -24.2 dB with a bandwidth of 294 MHz, confirming the expected trade-off between peak modulation efficiency and bandwidth for different numbers of IDT finger pairs.
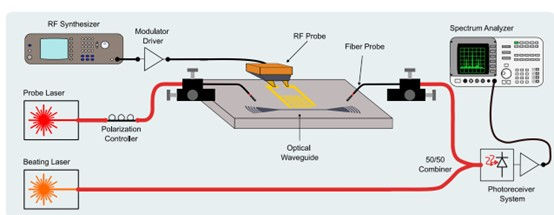
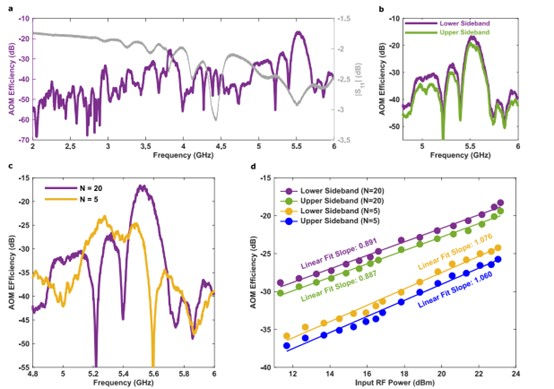
Performance Comparison and Significance
Table 1 compares the performance of the implemented AOM devices with other reported works. The device with 20 finger pairs achieves the highest reported modulation efficiency of -18.3 dB among silicon-based AOMs, while the device with 5 finger pairs exhibits more than an order-of-magnitude improvement in operation bandwidth compared to other reported works.
The developed AOM platform, implemented on a commercial silicon photonics process, paves the way for high-performance, high-yield CMOS-compatible AOMs, bringing advanced acoustic signal processing capabilities to silicon-CMOS-based photonic and electronic platforms. The monolithic integration of acousto-optic devices with other photonic and electronic components on the same chip enables numerous applications, including low-loss microwave signal processing, nonreciprocal light propagation, frequency comb generation, and broadband acousto-optic modulation.
Table 1. Comparison for silicon-based AOM platforms
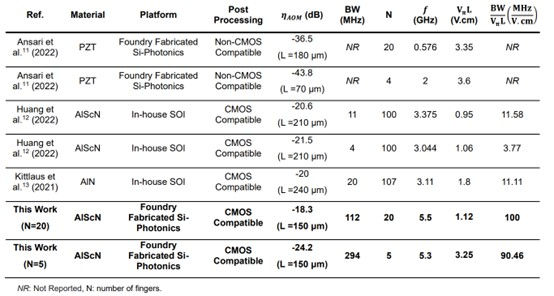
Potential Improvements and Future Prospects
The performance of the implemented transducer could be further improved by fabricating an acoustic reflector to realize a unidirectional transducer with higher efficiency. Additionally, a matching network can be added to the input of the transducer to improve the RF conversion efficiency.
The demonstrated integration of highly efficient acoustic devices on a CMOS-compatible commercial photonics process enables mass production of high-yield, low-cost acoustic, photonic, and CMOS devices on the same chip, resulting in advances in various applications, including sensing, imaging, signal processing, and computation.
Conclusion
This tutorial article has discussed the realization of a highly efficient, wideband AlScN-Si acousto-optic modulator on a commercially available silicon photonics process. The device structure, operating principle, fabrication process, and characterization methods have been explained in detail, along with the performance results and comparison with other reported works.
The monolithic integration of acousto-optic devices with photonic and electronic components on the same chip, enabled by this work, opens up exciting possibilities for low-cost, miniature microwave filters, true time delays, frequency combs, and other signal processors with advanced functionality offered by foundry-integrated photonic circuits.
Reference
[2] Erdil, Mertcan, Izhar, Yang Deng, Zichen Tang, Mohamad Hossein Idjadi, Farshid Ashtiani, Firooz Aflatouni and Roy Olsson. “Wideband, Efficient AlScN-Si Acousto-Optic Modulator in a Commercially Available Silicon Photonics Process.” (2024).
Comments