Preserving Ultra-Low Optical Loss in Silicon Nitride During Heterogeneous Laser Integration
- Latitude Design Systems
- Dec 4, 2024
- 3 min read
Introduction
Silicon nitride (Si3N4) photonics has emerged as a promising platform for various applications, including low-noise lasers, microresonator frequency combs, and photogalvanic-induced second harmonic generation. These applications rely on the ultra-low optical losses achievable in Si3N4 waveguides and resonators. However, integrating III-V lasers with Si3N4 can be challenging, as the additional processing steps may increase the optical loss of the Si3N4 structures. In this tutorial, we explore the investigation of Q degradation in low-loss Si3N4 microresonators during the heterogeneous laser integration process.
Fabrication Process
The fabrication process begins with the deposition of low-loss Si3N4 at a commercial CMOS foundry, followed by high-temperature annealing to drive out residual hydrogen and reduce optical loss. Silicon-on-insulator (SOI) and indium phosphide (InP) materials are then sequentially bonded, and their substrates are removed, leaving thin film layers. These layers are then processed through various backend steps, as illustrated in Figure 1.
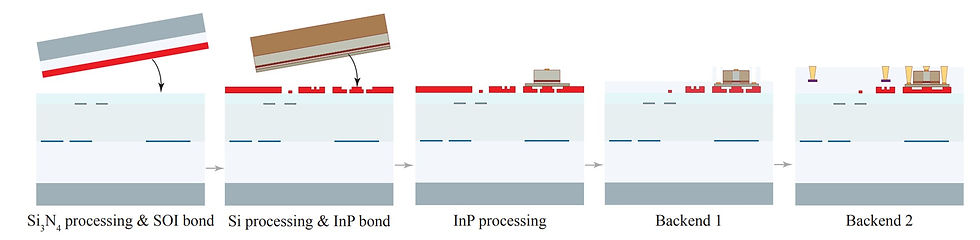
Q Measurements
To characterize the impact of each backend processing step on the optical loss of Si3N4 resonators, the resonator quality factor (Q) is measured after each step. The transmission spectra of the resonators are obtained by sweeping a calibrated external tunable laser, and the doublet resonances are fitted with a coupled-mode theory model to extract the loaded and intrinsic Qs.
Figure 2 presents the Q measurements after each backend step, including excess Si removal, oxide deposition and via etch, metal liftoffs, rapid thermal anneal (RTA), hydrogen implant, and probe metal liftoff. The rightmost panel shows the spectral averages of the intrinsic Q across three dies on a single wafer, demonstrating consistent performance.

Results and Discussion
The initial Q measurements after excess Si removal reveal intrinsic Qs near 100 million at 1550 nm. Subsequent processing steps, such as oxide deposition, via etching, and metal liftoffs, lead to a decrease in Q. However, thermal annealing steps, including oxide deposition at 250°C and RTA at 370°C, restore the Q to its original values. This reversible increase in loss is attributed to the population and depopulation of absorptive defect centers by above-bandgap optical exposure and thermal annealing, respectively.
Surprisingly, after hydrogen implantation and heater liftoff, the Q drops again, but it recovers slightly after probe metal liftoff. These observations suggest that various backend steps contribute to the loss increase, and further investigation is required to fully understand the reversible loss mechanism.
Previous work has cited UV irradiation from O2 plasma cleaning as the primary mechanism for populating defect centers in Si3N4, increasing absorption loss. However, in this work, the process included many more steps, necessitating further investigation.
Outlook
While the initial Si3N4 wafers from the same foundry run yielded Qi greater than 150 million at 1550 nm, further annealing may be required to recover similar values after the heterogeneous laser integration process. Additionally, since the InP received hydrogen implantation to form current channels, the annealing temperature and duration must be optimized to ensure that the laser gain is preserved while recovering the ultra-low loss in Si3N4.
Conclusion
This tutorial article highlights the importance of maintaining ultra-high Qs in Si3N4 resonators throughout the heterogeneous laser fabrication process. By characterizing the Q degradation and recovery at each backend step, the authors demonstrate the potential for preserving ultra-low losses down to 0.2 dB/m through thermal annealing. Numerous photonic technologies, such as narrow-linewidth lasers, microcombs, and second harmonic generation, rely on ultra-low losses in Si3N4 resonators. Therefore, uncovering loss mechanisms and maintaining these ultra-high Qs during heterogeneous laser integration is crucial for enabling low-SWaP field deployment of these next-generation devices.
Reference
[1] J. Guo, C. Xiang, W. Jin, J. Peters, M. Li, T. Morin, and J. Bowers, "Investigation of Q degradation in low-loss Si3N4 from heterogeneous laser integration," Department of Electrical and Computer Engineering, University of California, Santa Barbara, Santa Barbara, California, USA, 2024, pp. 1-6, doi: 979-8-3503-9404-7/24/$31.00 ©2024 IEEE.
Comments