Introduction
Photonic sensors based on laser absorption spectroscopy (LAS) are gaining increasing interest for applications such as process monitoring, environmental monitoring, and medicine. The development of powerful tunable mid-infrared lasers has enabled unambiguous detection of numerous substances at low concentrations, often with calibration-free operation. However, traditional mid-IR spectroscopy systems are complex, delicate, and expensive, limiting their potential for widespread deployment.
Photothermal spectroscopy (PTS) offers a promising alternative approach. In PTS, the modulated absorption of light by materials leads to modulated heating of the sample, causing thermal expansion that generates acoustic and thermal waves. The magnitude of the measured signal is directly proportional to the induced pressure/temperature change and the concentration of the sample, in contrast to LAS which relies on the Beer-Lambert absorption law.
This tutorial will introduce the concept of Photonic Integrated Circuit (PIC) assisted PTS, which combines the advantages of PTS with integrated photonics to create compact, low-cost, and high-performance spectroscopy systems.
Background
The basic principle behind PTS dates back to Alexander Graham Bell, who discovered that modulated absorption of light by materials leads to modulated heating of the sample, generating acoustic and thermal waves. In recent years, advances in interband and quantum cascade lasers have accelerated the growth of PTS techniques.
In a recent evolution of PTS, a Fabry-Perot (FP) interferometer has been used as a sensitive transducer for detecting induced refractive index changes in gas samples. The excitation laser beam is tuned to an absorption line of the target analyte and intensity modulated. The periodic excitation of the analyte molecules inside the FP etalon through absorption of laser radiation leads to a periodic modulation of the gas temperature due to photothermal heating, which causes a change in the refractive index. The etalon acts as a transducer, providing a signal when the target gas absorbs.
The photoinduced temperature change ΔT is given by the following equation, which highlights the attractiveness of miniaturization as the signal scales with the laser power and inversely with the volume of the beam that interacts with the sample V.
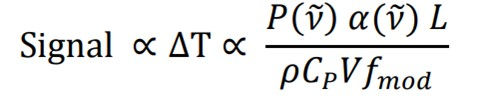
Where P(ν̃) is the laser power, α(ν̃) is the analyte linear absorption coefficient, L is the optical pathlength, ρ and Cp are the density and heat capacity of the sample, and fmod is the applied modulation frequency.
Experimental Setup
The authors demonstrate a photothermal sensor that uses a silicon nitride micro-ring resonator (MRR) refractive index sensor as a transducer, with a mid-infrared external cavity quantum cascade laser (EC-QCL) as the excitation source for a PTS measurement of water in ethanol, as shown in Figure 1.
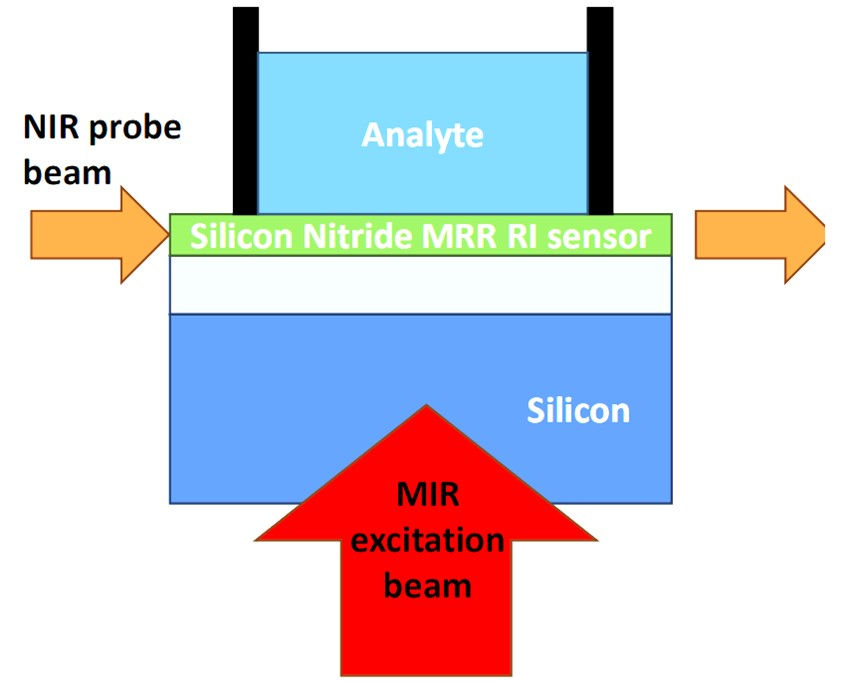
A tunable near-infrared (NIR) laser is set to the inflection point of a resonance of the MRR to maximize the wavelength shift sensitivity. The EC-QCL emits between 1560-1770 cm-1 (6410.26-5649.72 nm), covering the spectral region in which water in isopropanol has a strong absorption band.
The MRRs were fabricated using thermally oxidized silicon wafers with a SiN layer deposited by plasma-enhanced chemical vapor deposition (PECVD). The device layouts were patterned using electron beam lithography (EBL) and transferred to the SiN layer through inductively coupled plasma (ICP), as shown in Figure 2.

To perform the PTS experiment, the chip and analyte were illuminated from below by a Cassegrain reflector, ensuring a minimum beam waist at the focal point to concentrate the mid-IR radiation on the MRR (Figure 1). The excitation source was operated in pulse mode, with a pulse rate of 25 kHz.
The optical output signal at the telecoms wavelength from the MRR was coupled to an InGaAs photodetector. The signal from the detector was sent to a lock-in amplifier (LIA), with a time constant of 100 ms, taking the internal pulse trigger from the mid-IR laser as a reference, constituting the measured PTS signal.
Results and Discussion
An example spectrum obtained using this setup is shown in Figure 3. Savitzky-Golay filtering was performed to remove noise contribution from water vapor absorption.
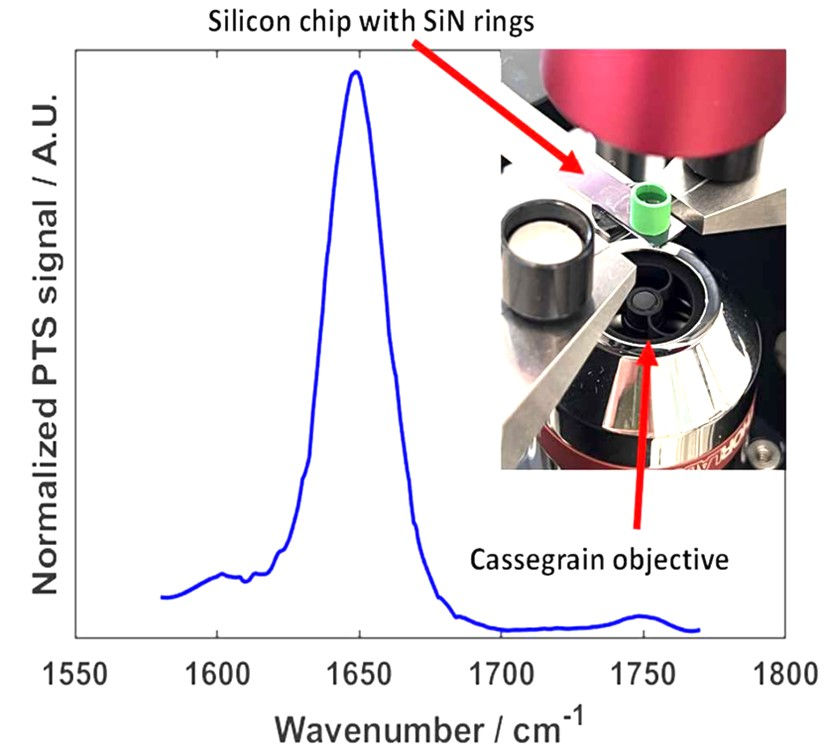
The authors have demonstrated that PIC-assisted PTS allows for sensor miniaturization by reducing the sample volume. The technique can cover a broad spectral region and can be applied to many analytes in both liquid and gas phases. Other transducers may be used, offering highly sensitive detection.
Summary
In this work, the authors reported a photothermal micro-ring resonator-based sensor for detecting water in isopropanol. The system is compact and exhibits short analysis times, taking a few seconds for a full scan. PIC-assisted PTS enables sensor miniaturization by reducing the sample volume while offering broad spectral coverage and applicability to various analytes in liquid and gas phases. This approach holds promise for creating low-cost, compact, and high-performance spectroscopy systems.
Reference
[1] G. Ricchiuti, A. Walsh, J. H. Mendoza-Castro, A. S. Vorobev, M. Kotlyar, S. Iadanza, M. Grande, B. Lendl, and L. O’Faolain, "Photonic Integrated Circuit Assisted Photothermal Spectroscopy," Institute of Chemical Technologies and Analytics, TU Wien, Vienna, Austria; Centre for Advanced Photonics and Process Analysis, Munster Technological University, Cork, Ireland; Tyndall National Institute, Cork, Ireland; Department of Electrical and Information Engineering, Polytechnic University of Bari, Bari, Italy; Laboratory of Quantum and Nanotechnologies Paul-Scherrer-Institut, Villigen PSI, Switzerland, 2024, pp. 1-6, doi: 979-8-3503-9404-7/24/$31.00 ©2024 IEEE.
コメント