Introduction
Quantum computers have the potential to solve currently intractable problems across fields like chemistry, materials science, cybersecurity, and more. However, today's quantum systems are small and unreliable, limited by errors from environmental interactions and control imperfections. To realize quantum's true potential, we need a scalable, fault-tolerant architecture for quantum computing.
This tutorial outlines the key lessons for designing such an architecture based on insights from Photonic Inc., a pioneer in silicon spin-photon quantum technologies. We'll explore how networked quantum modules that leverage integrated photonics can provide the scalability and reliability needed for large-scale quantum computing.
Lesson 1: All Platforms Will Evolve Towards Networked Quantum Modules
The technical requirements for error-free operation of qubits (quantum bits) are extremely stringent. Qubits must operate in strictly controlled environments or "boxes" which can only host up to around 1,000 qubits due to scaling limitations.
To build more powerful quantum computers, we need to network multiple smaller quantum modules together, similar to how classical computers network multiple processor cores. This horizontal scaling approach circumvents the qubit count limits of individual modules.
Photons of telecom wavelength light are extremely reliable qubits that can transmit quantum information between modules at room temperature using conventional fiber optic cables. By using telecom photons to link multiple quantum processor modules via optical input/output (I/O) ports, we can build arbitrarily large networked quantum computers.
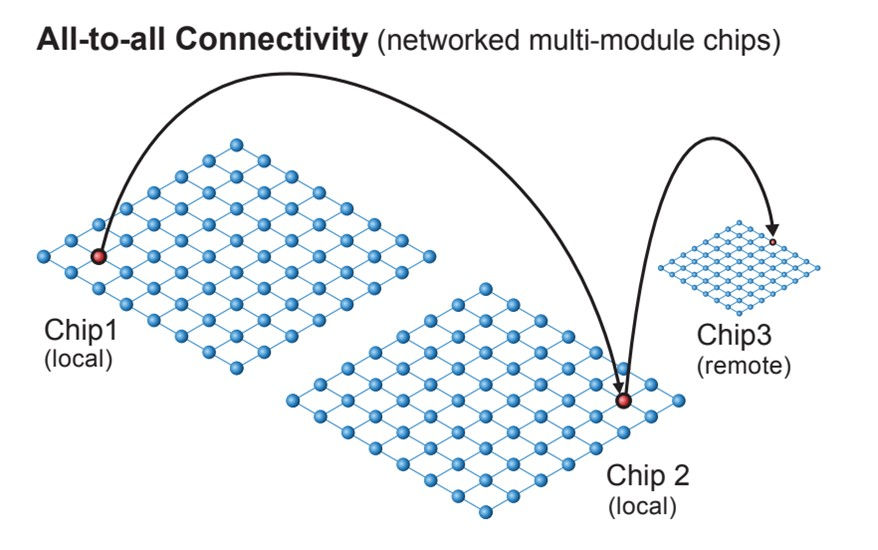
Lesson 2: I/O Quantity Matters - We Want Quantum I/O for Each Qubit
A key difference between classical and quantum computers is the enormous number of I/O ports required for quantum systems. In many quantum algorithms, every physical qubit must directly link to partner qubits in other modules to perform reliable quantum logic operations.
This level of connectivity requires vastly more I/O than classical computing networks. Having a dedicated optical I/O per qubit is ideal to maximize performance, as anything less will create a throttling bottleneck. Scalable quantum systems must be designed around maximizing their core I/O capability from the start.
Lesson 3: I/O Quality Matters - Efficient Photon Collection & Transmission
Simply having optical I/O ports is not enough - we must efficiently collect and transmit photons through these ports. Some qubits like Photonic's silicon spin qubits have a "built-in" optical interface that directly connects to telecom fiber without lossy wavelength conversion.
Other qubit types require low-efficiency tranducers to convert to telecom wavelengths, adding noise and slowing computations by 10x. Vacuum quantum systems also suffer from poor photon collection efficiencies as low as 5%, another 20x slowdown.
Solid-state spin qubits like Photonic's can overcome both issues. Their optical cavities inherently guide photons into and out of fiber optic modes, enabling efficient telecom photon I/O without conversion loss or collection issues.
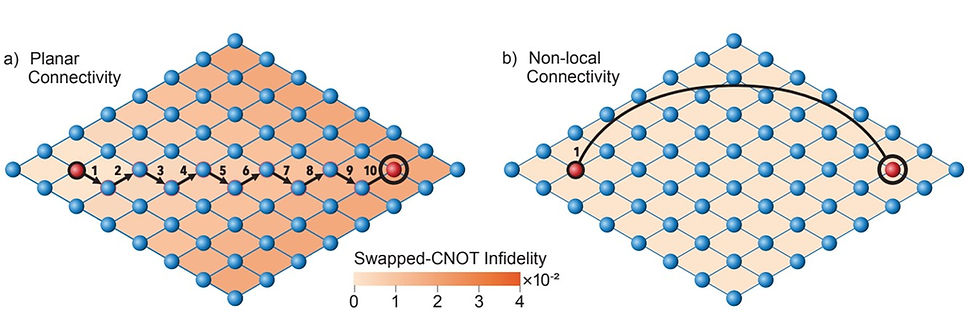
Lesson 4: Connectivity is Key for Scalability and Fault-Tolerance
A networked quantum architecture should leverage the ability of photons to directly transmit qubits between any modules. This "non-local connectivity" eliminates inefficient qubit routing operations within modules and enables highly efficient error correction.
Non-local connectivity allows quantum error correction to be implemented with up to 100x fewer physical qubits compared to planar nearest-neighbor architectures. This drastically reduces the required engineering precision and leads to more reliable, scalable quantum computing.
Photonic's Scalable Networked Quantum Architecture
Photonic Inc. has developed a unique quantum computing platform built around silicon-based spin qubits and integrated photonics that directly addresses the scalability lessons outlined above:
Networkability & Horizontal Scaling: Photonic's spin qubits are based on defects in silicon called T centers that emit at telecom wavelengths. This allows fiber optic networking of multiple quantum processor chips for horizontal scaling.
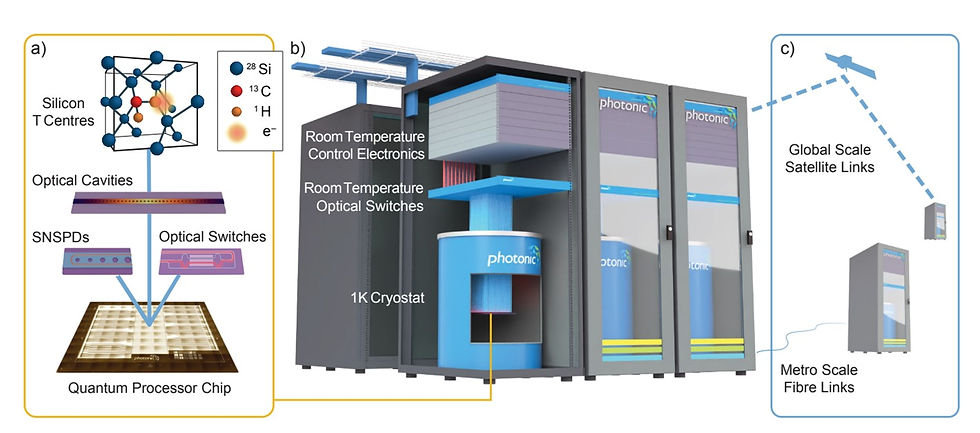
I/O Quantity: Photonic's architecture achieves non-local connectivity by using integrated photonic waveguides and switches to provide each qubit with a dedicated optical I/O.
I/O Quality: The telecom emission of T centers avoids lossy wavelength conversion. And their integrated solid-state platform inherently guides photons to/from fiber optic modes for efficient collection and transmission.
Connectivity & Fault-Tolerance: With non-local connectivity between all qubits, Photonic's approach can leverage highly efficient fault-tolerance techniques requiring far fewer physical qubits.
Realizing the Game-Changing Potential of Quantum
While the technical hurdles for reliable, scalable quantum computing are high, innovations like Photonic's are showing there is a viable path forward. By applying key architectural lessons around networking qubits and leveraging integrated photonics, we can build large-scale quantum systems that can tackle problems currently beyond our capabilities.
Quantum computing has immense potential to drive breakthroughs across chemistry, materials science, optimization, cryptography, and more. A scalable, fault-tolerant quantum architecture networked via telecom photonics may be the key that finally unlocks this world-changing technological revolution.
Reference
[2] S. Simmons, "What Could Networks of Quantum Supercomputers Look Like?" White Paper, Photonic Inc., November 8, 2023. [Online]. Available: www.photonic.com
Comments