Terence S.-Y. Chen
Latitude Design Systems
Abstract
This paper reviews the recent perspective by McMahon on harnessing the physical features of optics to potentially achieve advantages in specialized optical computing architectures. Optics has seen a resurgence of interest for computing, especially for neural networks. However, general purpose optical computing faces substantial challenges compared to state-of-the-art digital electronics.
This paper summarizes McMahon's analysis of 11 inherent features of optics that could confer benefits, including massive bandwidth, dissipationless dynamics, and spatial parallelism. Careful engineering is required to exploit these features while avoiding pitfalls from input/output bottlenecks and interconnects. Promising applications for specialized analog optical computers include neural network inference and combinatorial optimization. But significant work remains to build competitive holistic systems. This review distills McMahon's insights on the physics of optical computing and assess the field's prospects based on fundamental capabilities and limitations of optics.
Introduction
The promise and challenge of optical computing has seen rising interest in recent years, both in research and commercial efforts. Optics offers potential advantages stemming from fundamental physics, but realizing competitive systems requires overcoming daunting challenges. McMahon [1] provides an invaluable perspective on this landscape by enumerating 11 key features of optics that could confer benefits, while analyzing the architectural and engineering obstacles that must be tackled. This paper reviews McMahon’s analysis to elucidate the physics enabling optical computing and assess its potential through the lens of fundamental capabilities and limitations.
McMahon outlines why optical computing is unlikely to replace general purpose digital electronics in the near future. But specialized analog optical computers may compete for tasks like neural network inference if harnessed judiciously. This paper summarizes McMahon’s enumerated optical features and how they could be exploited. Architectural considerations and design strategies to mitigate pitfalls are discussed. Promising application domains are highlighted where optics may offer a path to outperforming electronics by combining multiple physical advantages. Finally, remaining challenges identified by McMahon are examined.
Background and Purpose
Interest in optical computing has endured since the 1960s, including pioneering work on optical neural networks. But progress has seen ups and downs over the decades. Recently, academic and industrial efforts have surged, especially on specialized optical neural network accelerators.
McMahon aims to provide a systematic analysis of whether, why, and how optics could confer speed or efficiency benefits for computing compared to electronics. Key goals are enumerating the salient features of optics that can be harnessed, clarifying misconceptions about sources of optical advantage, identifying promising applications and holistic system design strategies, and elucidating remaining challenges. The analysis focuses on whether specialty analog optical computers can compete with state-of-the-art digital electronics.
Optical Features for Computing
McMahon delineates 11 salient features of optics that could confer advantages:
Extremely high bandwidth, enabling massive parallelism in frequency modes.
Fast dynamics approaching the bandwidth limit, resulting in high speed.
Spatial parallelism with large numbers of modes, especially in 3D.
Nearly dissipationless linear and nonlinear propagation for low-energy computing.
Low-loss transmission over long distances, reducing communication costs.
Interference, allowing operations like Fourier transforms.
Nonlinear optics for complex nonlinear functions.
Entanglement for quantum computing.
Slow light enhancing light-matter interactions.
Stability owing to reduced defects compared to nanoscale electronics.
Reconfigurability, programmability, and interconnectivity.
These provide diverse mechanisms for improved performance and efficiency. But engineering solutions holistically is crucial to harnessing them effectively while avoiding pitfalls.
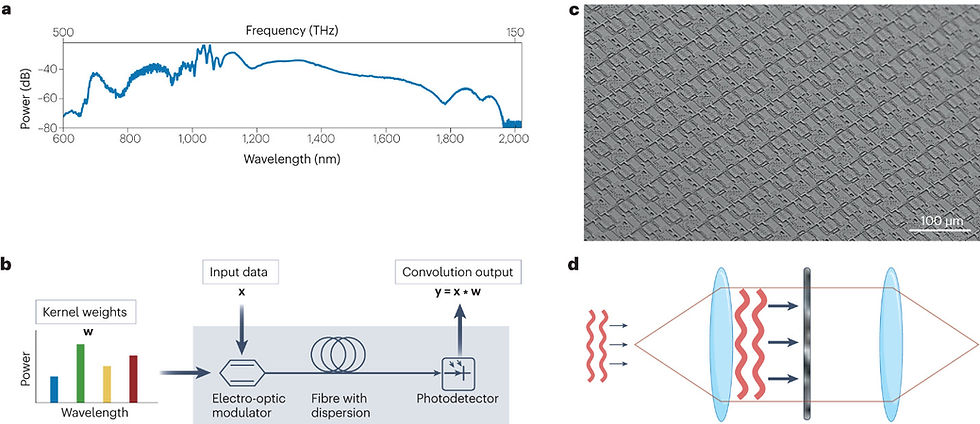
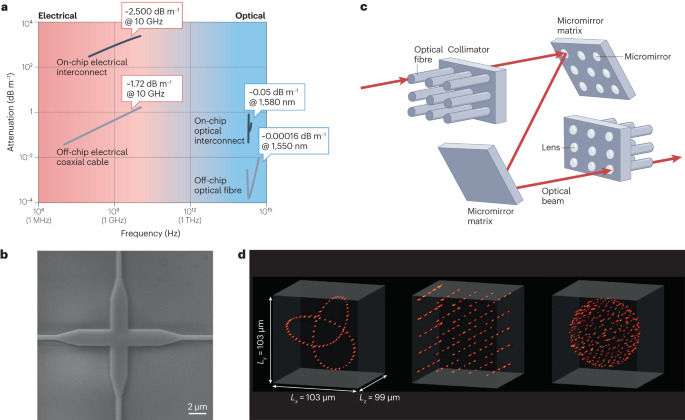
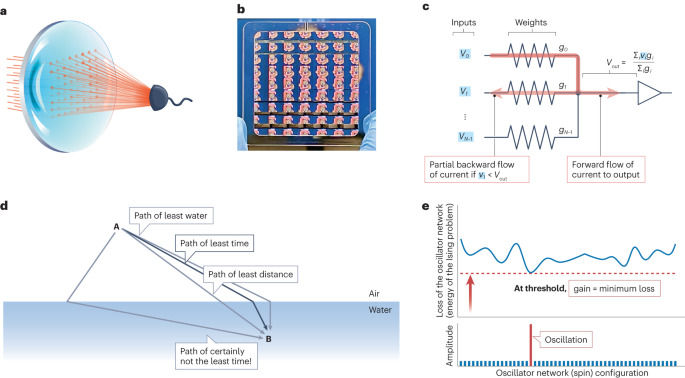
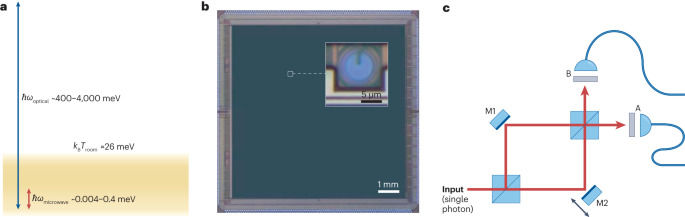
Key Optical Advantages
McMahon emphasizes that the oft-cited benefit of fast speed of light alone does not confer advantages. Rather, the potential stems from the ensemble of optical features. Massive bandwidth enables minimal delay and parallelism. Spatial parallelism allows simultaneous operations orders of magnitude above electronics. Dissipationless propagation incurs almost no energy costs for linear and nonlinear computation. Additional advantages arise from characteristics like low-loss transmission, interference, nonlinearity, and stability against defects.
Architectural Considerations
McMahon notes architectures like analog systolic arrays for both electronics and optics can enable easier comparison by isolating physical distinctions. Optical computers should minimize transduction steps between optical and electronic domains to combat conversion overhead. Hybrid systems combining optical and electronic processing may optimize tradeoffs. Photonic integrated circuits can provide miniaturization and density improvements over bulk optics.
Promising Applications
McMahon identifies key application areas as neural network inference, combinatorial optimization, scientific computing, and cryptography. These involve heavy matrix-vector multiplication workloads well-suited to analog optical implementations. Accepting limited precision and errors provides tolerance for analog noise. Optical correlators also hold promise as commercial products. Optics is unlikely to displace digital electronics for general purpose computing necessitating total accuracy.
Remaining Challenges
Despite great progress, optical computing faces steep challenges. Input/output bottlenecks and costs of optical-electronic conversion remain concerns. Interconnects between components are limited compared to electronics. Engineering economically manufacturable systems requires further materials, device, and integration developments.
Implementation Technologies
McMahon notes photonic integrated circuits can provide miniaturization over bulk optics. Silicon photonics is a leading integration approach, with companies like AIM Photonics producing low-loss silicon nitride waveguides. This enables chip-scale optical processing. Nonlinear optics in materials like III-V semiconductors could potentially be useful for optical computing applications requiring nonlinearity. However, McMahon does not delve deeply into assessing specific material platforms, focusing more on conceptual advantages.
Analog Optical Computing vs. Digital Electronics
McMahon argues that general purpose optical computing for error-free, high precision applications remains inferior to digital electronics. But analog optical computing shows promise on specialized tasks where approximation is acceptable, like inference. Digital electronics have benefited enormously from engineering efforts on transistors and logic. But analog optics can exploit massive parallelism and fast switching beyond electronics on suitable workloads while tolerating low precision. Overall capability is still constrained by interconnect and input/output bottlenecks.
Key Findings and Insights
McMahon notes renewed interest in optical computing, particularly for neural networks with high computational demands. Optics likely won't replace general digital systems but may have advantages on specialized workloads. He enumerates 11 key optical features that could confer benefits if harnessed holistically, including bandwidth, dissipationless operation, and spatial parallelism. Optical neural network accelerators may compete with analog electronics. Optical matrix-vector multiplications can beat fundamental electronic limits. But major challenges remain like input/output bandwidth and interconnects.
Conclusion
This review synthesized McMahon's perspective highlighting 11 salient features of optics that could enable specialized optical computers to compete with state-of-the-art electronics for tasks amenable to analog processing if harnessed judiciously. Significant engineering obstacles remain, especially regarding input/output bandwidth and interconnects. But the fundamental physics of optics provides a foundation to build upon for applications like neural inference. McMahon delivers an invaluable, comprehensive analysis of the promise and challenges of optical computing grounded in fundamental capabilities and limitations.
Reference
[1] McMahon, P.L. The physics of optical computing. Nat Rev Phys (2023). https://doi.org/10.1038/s42254-023-00645-5
Comments