Introduction
Nonlinear optics lies at the heart of photonics, enabling a wide range of applications from frequency conversion to quantum light generation. Lithium niobate (LN) has long been recognized as an important nonlinear optical material due to its large second-order nonlinear coefficient. With the development of lithium-niobate-on-insulator (LNOI) nanophotonics in recent years, new opportunities have emerged to harness the nonlinear optical properties of LN in compact, integrated devices.
This article will provide an overview of recent advances in nonlinear optics using LNOI nanophotonic structures. We will cover key nonlinear optical phenomena including second harmonic generation (SHG), sum-frequency generation (SFG), difference-frequency generation (DFG), four-wave mixing (FWM), Raman scattering, frequency comb generation, and optomechanics. The tutorial will discuss various phase-matching schemes, device designs, and experimental demonstrations that have enabled high-efficiency nonlinear interactions in LNOI devices.
Second Harmonic Generation
Second harmonic generation is one of the most basic and widely studied nonlinear optical processes. In SHG, two photons at the fundamental frequency combine to generate a single photon at twice the frequency. Efficient SHG requires phase-matching between the interacting waves. In LNOI nanophotonic structures, several phase-matching schemes have been demonstrated:
Modal Phase-Matching:
This approach utilizes the modal dispersion in waveguides to achieve phase-matching between different spatial modes at the fundamental and second harmonic frequencies. Figure 1 shows an example of modal phase-matched SHG in an LNOI microring resonator:
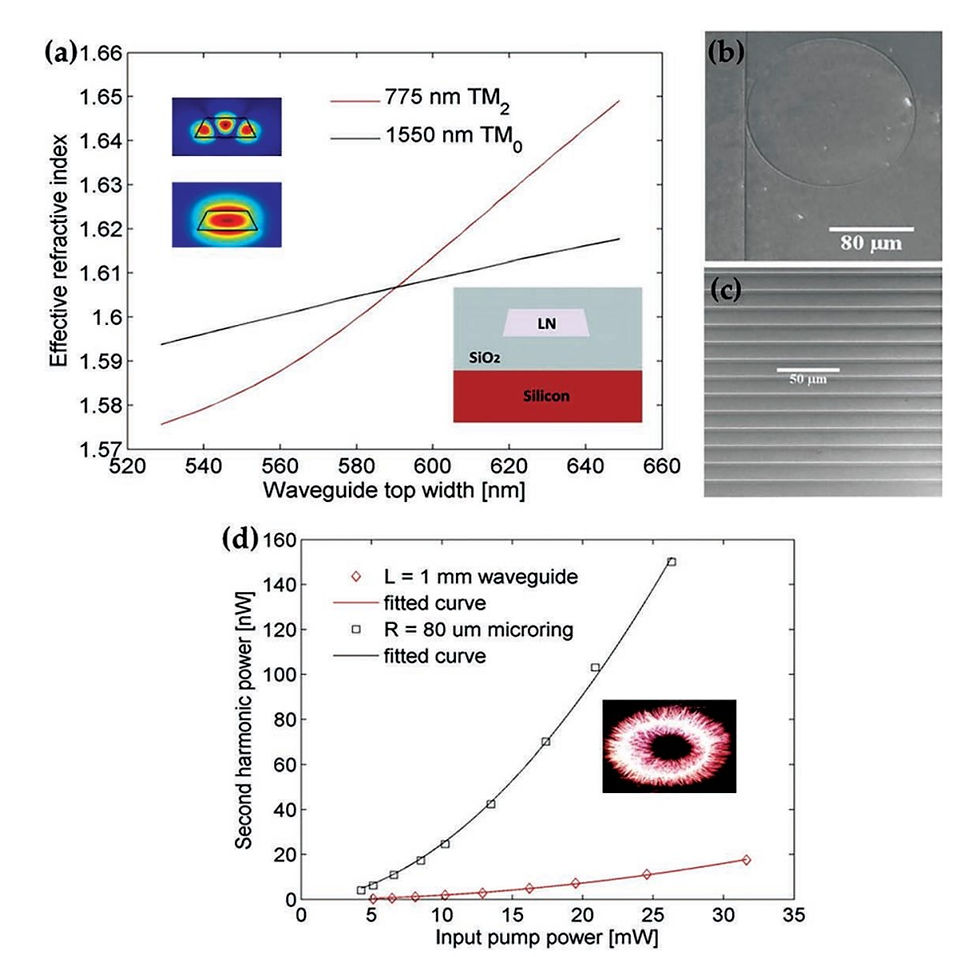
The fundamental TE mode at 1550 nm is phase-matched to the TM20 mode at 775 nm by carefully engineering the waveguide dimensions. This enables efficient SHG with a normalized conversion efficiency of ~2.22 x 10^-6 W^-1.
Quasi-Phase-Matching:
Periodic poling of the LN crystal allows quasi-phase-matching (QPM) by periodically inverting the sign of the nonlinear coefficient. Figure 2 shows SHG in a periodically poled LNOI (PPLN) waveguide:

The PPLN waveguide achieves an absolute SHG conversion efficiency of 53%, close to the theoretical limit. QPM provides flexibility in choosing the interacting wavelengths and polarizations.
Other approaches like cyclic phase-matching in whispering gallery mode resonators have also been demonstrated. The strong optical confinement and high quality factors of LNOI resonators enable SHG with very low pump powers, down to the microwatt level.
Sum and Difference Frequency Generation
Sum-frequency generation (SFG) and difference-frequency generation (DFG) are three-wave mixing processes involving two input frequencies and one output frequency. These processes enable flexible frequency conversion and are useful for applications like wavelength conversion in optical communications.
Figure 3 shows an example of SFG in an LNOI microdisk resonator:
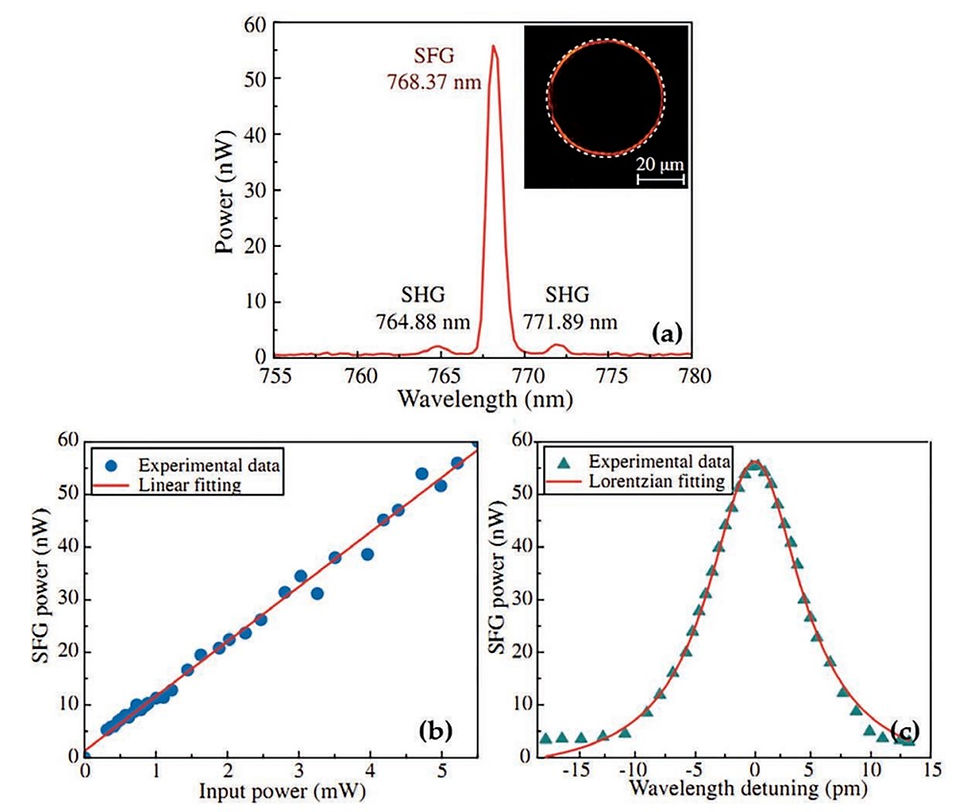
Two pump lasers at 1529.76 nm and 1543.78 nm generate an SFG signal at 768.37 nm through modal phase-matching. The process shows a linear dependence on the pump powers, with a normalized conversion efficiency of ~2.22 x 10^-6 W^-1.
DFG has also been demonstrated in PPLN waveguides on LNOI. Figure 4 shows broadband DFG covering the entire telecom C-band:

With a fixed signal at 1400 nm and a tunable pump from 730-740 nm, idler wavelengths from 1520-1570 nm are generated. A high normalized DFG efficiency of 10,300% W^-1 cm^-2 is achieved.
Third-Order Nonlinear Effects
While LN is known for its strong second-order nonlinearity, third-order nonlinear effects can also be observed in LNOI devices. These include:
Cascaded Third Harmonic Generation:
THG can occur through cascaded second-order processes, where the second harmonic further interacts with the fundamental to generate the third harmonic. Figure 5 shows cascaded THG in an LNOI microdisk:
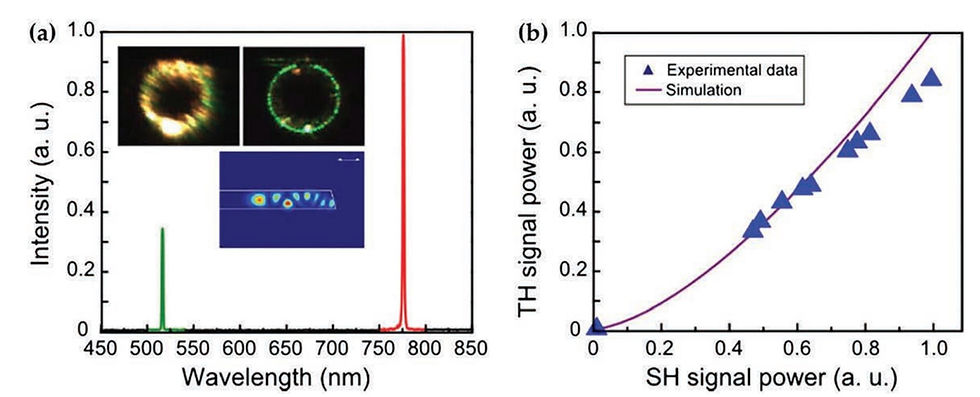
Both SHG at 775.7 nm and THG at 516.5 nm are observed when pumping at 1551.4 nm. The THG power shows a cubic dependence on the fundamental power, as expected for a cascaded process.
Four-Wave Mixing:
FWM is a third-order nonlinear process where two pump photons generate signal and idler photons at new frequencies. Figure 6 shows efficient FWM near the second harmonic wavelength in an LNOI photonic molecule:
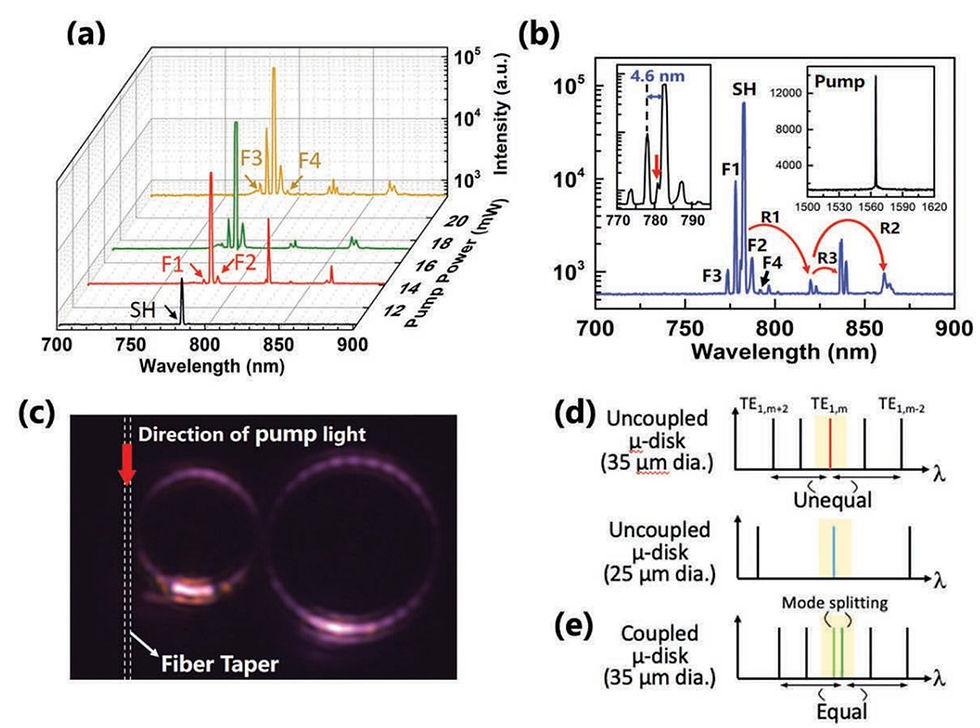
When pumping at 1565.46 nm, FWM sidebands are generated symmetrically around the second harmonic at 782.73 nm. The process shows a high conversion efficiency of ~14% at only milliwatt-level second harmonic powers.
Raman Scattering:
Stimulated Raman scattering has been observed in high-Q LNOI resonators, enabled by the strong vibrational modes of the LN crystal. This has allowed generation of broadband Raman frequency combs.
Frequency Comb Generation
Optical frequency combs are important tools for precision metrology, spectroscopy, and optical communications. LNOI microresonators have emerged as a promising platform for on-chip comb generation through several mechanisms:
Electro-Optic Combs:
The strong electro-optic effect in LN enables comb generation through phase modulation. Figure 7 shows an electro-optic comb generator based on an LNOI racetrack resonator:
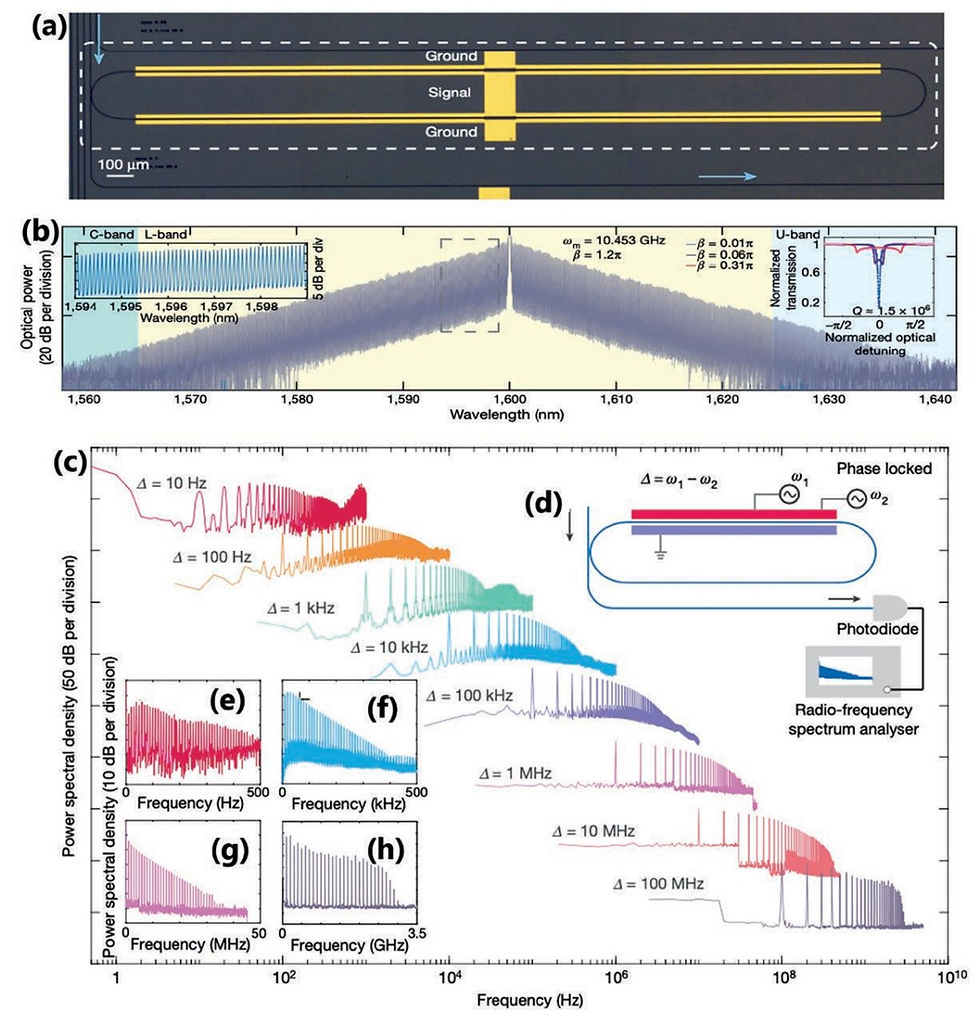
Microwave modulation at 10.453 GHz generates a comb spanning 80 nm with over 900 comb lines. The comb spacing is reconfigurable by tuning the modulation frequency.
Kerr Combs:
Despite the relatively weak Kerr nonlinearity of LN, careful dispersion engineering has enabled demonstration of Kerr frequency combs and soliton states. Figure 8 shows single and multi-soliton states in an LNOI microring:
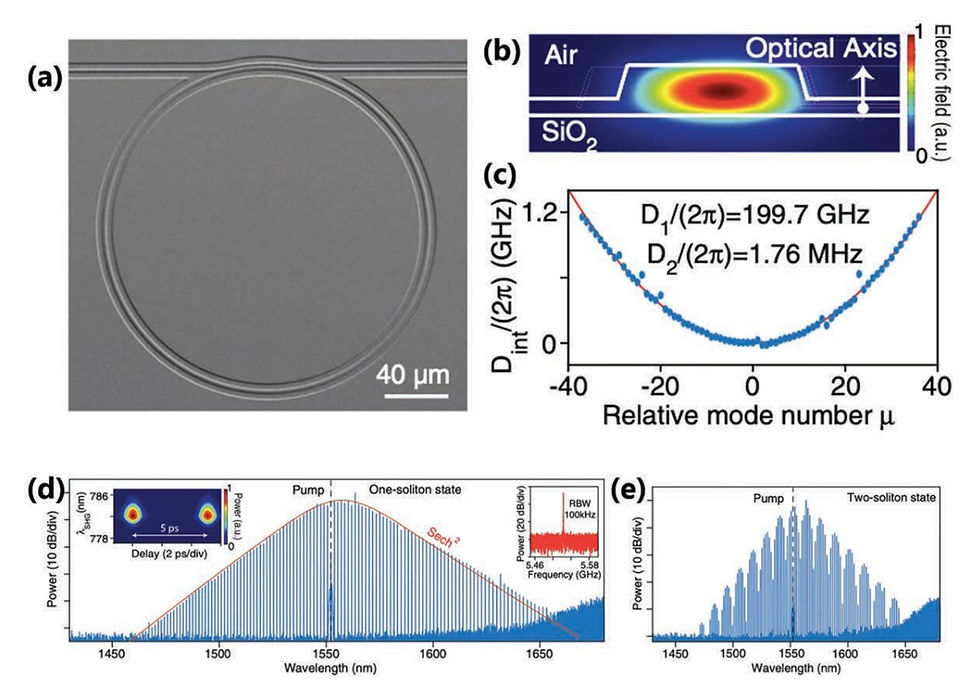
Soliton combs with smooth spectral envelopes are generated by pump laser detuning. The interplay between the photorefractive effect and Kerr nonlinearity in LN enables unique features like bidirectional soliton switching.
Raman Combs:
As mentioned earlier, stimulated Raman scattering in LNOI resonators can also lead to broadband comb generation, leveraging the Raman gain of the LN crystal.
The ability to generate combs through multiple mechanisms (electro-optic, Kerr, Raman) in a single integrated platform is a unique advantage of LNOI.
Optomechanics
The high optical quality factors and small mode volumes achievable in LNOI resonators have enabled observation of optomechanical effects. Figure 9 shows an LNOI optomechanical resonator:
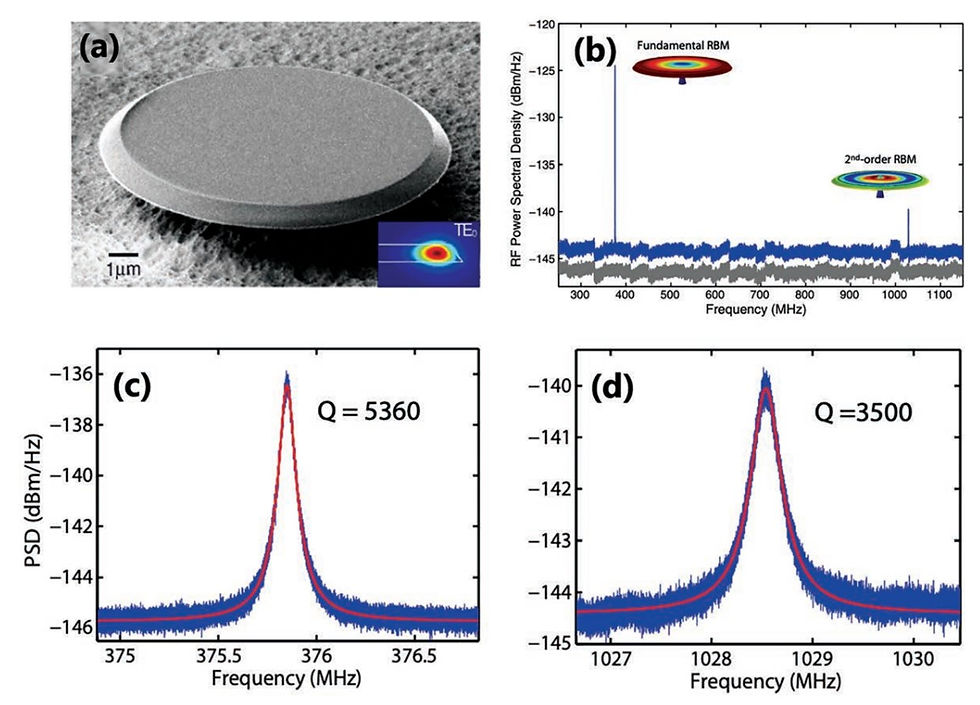
Radial breathing mechanical modes at hundreds of MHz frequencies are observed through optical readout. The devices show high mechanical quality factors exceeding 5000, with frequency-quality products over 10^12 Hz.
The combination of optomechanical, electro-optic, and nonlinear optical effects in a single LNOI platform opens up exciting possibilities for hybrid photonic-phononic devices.
Conclusion and Outlook
This tutorial has provided an overview of recent advances in nonlinear optics using lithium niobate nanophotonic structures. Key achievements include:
High-efficiency second harmonic generation approaching 100% conversion
Broadband wavelength conversion through sum and difference frequency generation
Kerr, electro-optic, and Raman frequency comb generation
Observation of optomechanical effects with high mechanical quality factors
The combination of strong optical confinement, low propagation losses, and the intrinsic nonlinear optical properties of lithium niobate has enabled nonlinear optical interactions at unprecedented low power levels. Many nonlinear optical processes that traditionally required bulk crystals or high-power pulsed lasers can now be realized in compact, integrated devices operating with continuous-wave pumping at milliwatt power levels.
Looking ahead, there are several promising directions for further development:
Improved fabrication techniques to realize ultra-high Q resonators (>10^7) and low-loss waveguides, further enhancing nonlinear efficiencies.
Advanced dispersion engineering and resonator designs to enable even broader bandwidth frequency conversion and comb generation.
Heterogeneous integration of LNOI with other photonic platforms like silicon and III-V semiconductors to create hybrid devices with enhanced functionality.
Exploration of quantum optical effects like squeezed light generation and entangled photon pair sources using LNOI nanophotonic structures.
Development of fully integrated nonlinear optical systems incorporating on-chip lasers, modulators, and detectors alongside LNOI nonlinear elements.
The rapid progress in LNOI nonlinear optics over the past few years suggests that many of these goals are within reach. As fabrication techniques mature and device designs are optimized, we can expect LNOI to play an increasingly important role in both classical and quantum photonic technologies. The ability to harness strong second-order and third-order nonlinearities alongside electro-optic and optomechanical effects in a single integrated platform makes LNOI a uniquely versatile material for next-generation photonic devices.
Reference
[1] Y. Cheng, "Lithium Niobate Nanophotonics," Jenny Stanford Publishing, 2021.
Comments