Introduction
The ever-increasing demand for high-speed communication networks has driven the rapid development of silicon photonics, which offers advantages such as small size, high bandwidth, low cost, and low power consumption. To enhance transmission capacity and spectral efficiency, techniques like mode division multiplexing (MDM), wavelength division multiplexing (WDM), and orbital angular momentum (OAM) multiplexing have been proposed on the silicon platform. In this tutorial, we explore a novel on-chip optical power splitter on the silicon-on-insulator (SOI) platform that utilizes thermo-optic phase shifters and inverse design to achieve arbitrary mode selection between TE0, TE1, and TE2 modes at the output ports.
Device Design
The proposed optical power splitter, as illustrated in Fig. 1, consists of a 1 × 6 multi-mode interferometer (MMI), two 3 × 3 MMI switches, and a 6 × 2 inverse design region.
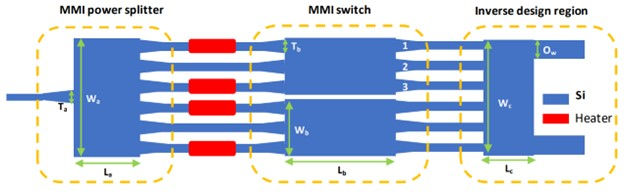
The input TE0 mode optical signal is first split into six channels using the 1 × 6 MMI. Metal heaters control the optical phases of these six arms via the thermo-optic effect. The signals then pass through two 3 × 3 MMI switches, whose designs are based on previous work [6]. Finally, the signals enter the inverse design region for mode conversion to achieve the desired TE0, TE1, or TE2 modes at the output ports.
Inverse Design Region
The inverse design region, shown in Fig. 2(a), utilizes the Direct Binary Search (DBS) algorithm to reduce the mode conversion area. The device is mirrored both vertically and horizontally, with dimensions of Wc = 7.2 μm, Lc = 4.8 μm, and Ow = 1.35 μm. It is fully etched, with etching performed in a square size of 120 nm × 120 nm, as depicted in Fig. 2(b), satisfying the minimum size requirement for fabrication foundries like IMEC®.
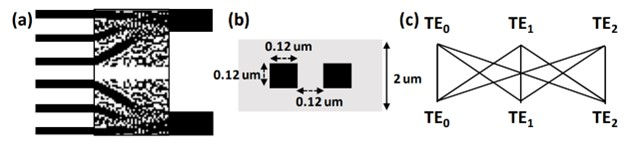
By controlling the input channels to the inverse design region, the device can output different modes, as illustrated in Fig. 2(c). This capability to selectively distribute power between TE0, TE1, and TE2 modes at each output port makes the proposed optical power splitter versatile for various integrated photonic applications and system-on-chip signal processing.
Simulation Results
The transmission performance of the different components in the optical power splitter was evaluated using finite-difference time-domain (FDTD) simulations in Lumerical®. The 1 × 6 MMI provides six equal power outputs, and the phase control is achieved via metal heaters. Table I shows the phase reference values applied to the top, middle, and bottom waveguides (WGs) on the left side of the 3 × 3 MMI switch to produce optical outputs at the top, middle, and bottom WGs on the right side, respectively.
Table I. 3 × 3 MMI switch phase reference
Top WG (Left) | Middle WG (Left) | Bottom WG (Left) | |
Phase | π | 0 | π/3 |
-π/3 | 0 | -π/3 | |
-π/3 | 0 | π |
Figures 3(a)-(c) show the FDTD simulation results of the 3 × 3 MMI switch when the signals are output at the top, middle, and bottom WGs, respectively. The simulated crosstalk values for the top, middle, and bottom WGs are –27 dB, –21 dB, and –27 dB, respectively, as shown in Figs. 3(d)-(f).

Figure 4 presents the FDTD simulations and modal transmission spectra for the inverse design region. Figures 4(a)-(c) show that the TE0 modes input into the 3rd and 4th, 2nd and 5th, and 1st and 6th input WGs of the 6 × 2 inverse design region will output two TE2, TE1, and TE0 modes, respectively. Due to the necessity of square etching, higher penetration loss is observed in the inverse design region, with peak losses of –4.4 dB, –6.8 dB, and –5.15 dB at 1550 nm for the TE0, TE1, and TE2 output cases, respectively, as shown in Figs. 4(d)-(f).

Conclusion
In this tutorial, we explored a miniaturized on-chip optical power splitter on the SOI platform that utilizes thermo-optic phase shifters and inverse design to achieve arbitrary mode selection between TE0, TE1, and TE2 modes at the output ports. The proposed design, composed of a 1 × 6 MMI, two 3 × 3 MMI switches, and a 6 × 2 inverse design region, offers flexibility in selectively distributing power among different modes. This versatile device has potential applications in integrated photonics and system-on-chip signal processing.
Reference
[2] Y.-Z. Lin, C.-W. Chow, and C.-H. Yeh, "Miniaturized Optical Power Splitter with Arbitrary Output Modes Using Inverse Design and Active Phase Shifters," Department of Photonics, National Yang Ming Chiao Tung University, Hsinchu 30010, Taiwan; Department of Photonics, Feng Chia University, Seatwen, Taichung 40724, Taiwan, 2024, pp. 1-6, doi: 979-8-3503-9404-7/24/$31.00 ©2024 IEEE.
Comments