Introduction
Lithium niobate (LN) has long been prized for its strong electro-optic (EO) effect, making it an ideal material for optical modulators and other photonic devices. However, traditional LN devices based on bulk crystals or weakly-confined waveguides suffer from large footprints and high power requirements. The emerging field of lithium-niobate-on-insulator (LNOI) photonics aims to overcome these limitations by combining the excellent EO properties of LN with nanoscale waveguides and resonators. This tutorial will explore recent advances in integrated EO devices on the LNOI platform, including high-Q microresonators, ultra-fast modulators, and novel nonlinear photonic components.
Electro-Optic Tuning of LNOI Microresonators
Microring and microdisk resonators are key building blocks for integrated photonics. The ability to precisely tune their resonance frequencies via the EO effect makes LNOI an attractive platform for reconfigurable devices.
Early work by Guarino et al. in 2007 demonstrated EO tuning of an LNOI microring with a modest efficiency of 0.14 GHz/V. The device structure is shown in Fig. 1:
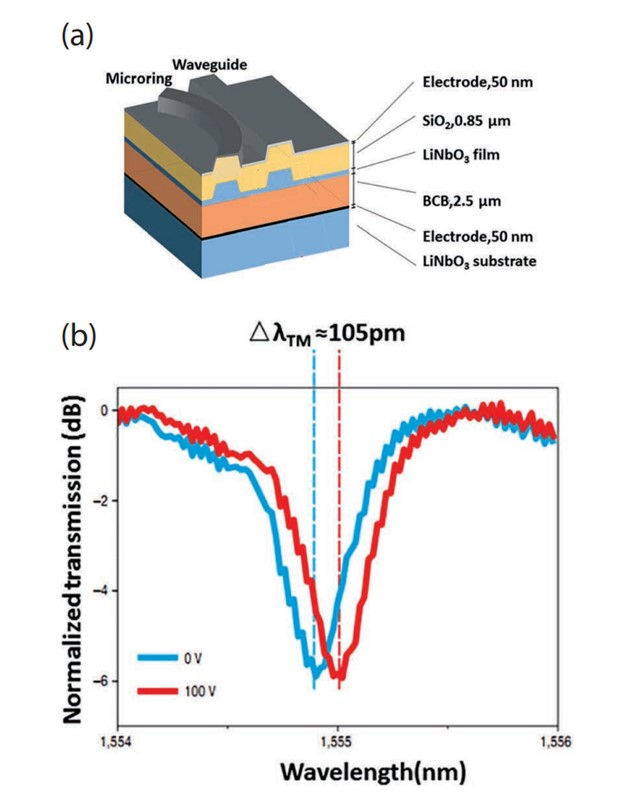
More recent efforts have dramatically improved performance through optimized designs. Wang et al. achieved a tuning efficiency of 3.0 GHz/V in 2015 using a high-Q microdisk resonator. By integrating electrodes with small gaps directly on the chip, Fang et al. further increased the efficiency to 29.2 pm/V (3.6 GHz/V) in 2020.
A key breakthrough was the development of sophisticated electrode designs to maximize the overlap between the applied electric field and the optical mode. Figure 2 shows an example of such an optimized structure:
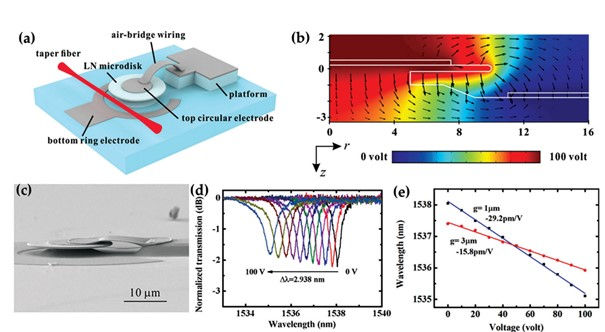
The circular electrodes concentrate the electric field along the microdisk rim, aligning it with the optical whispering gallery mode. This design achieved a record tuning efficiency of 29.2 pm/V.
High-Speed LNOI Modulators
Optical modulators are critical components for fiber-optic communications and photonic information processing. LNOI technology enables modulators with unprecedented combinations of speed, efficiency, and compact footprint.
Wang et al. demonstrated a Mach-Zehnder interferometer (MZI) modulator in 2018 capable of 100 Gbps data transmission with a half-wave voltage (V_π) of only 1.4 V. The device structure is shown in Fig. 3:

The tightly confined ridge waveguides allow for small electrode gaps, dramatically reducing the drive voltage compared to conventional LN modulators. The traveling-wave electrode design ensures velocity matching between the RF and optical signals, enabling ultra-wide bandwidth operation.
He et al. reported a similar high-performance modulator based on a hybrid Si/LN platform in 2019. Their device achieved 100 Gbps on-off keying and 112 Gbps PAM-4 modulation with a total insertion loss of only 2.5 dB. Figure 4 shows eye diagrams demonstrating the high-speed performance:
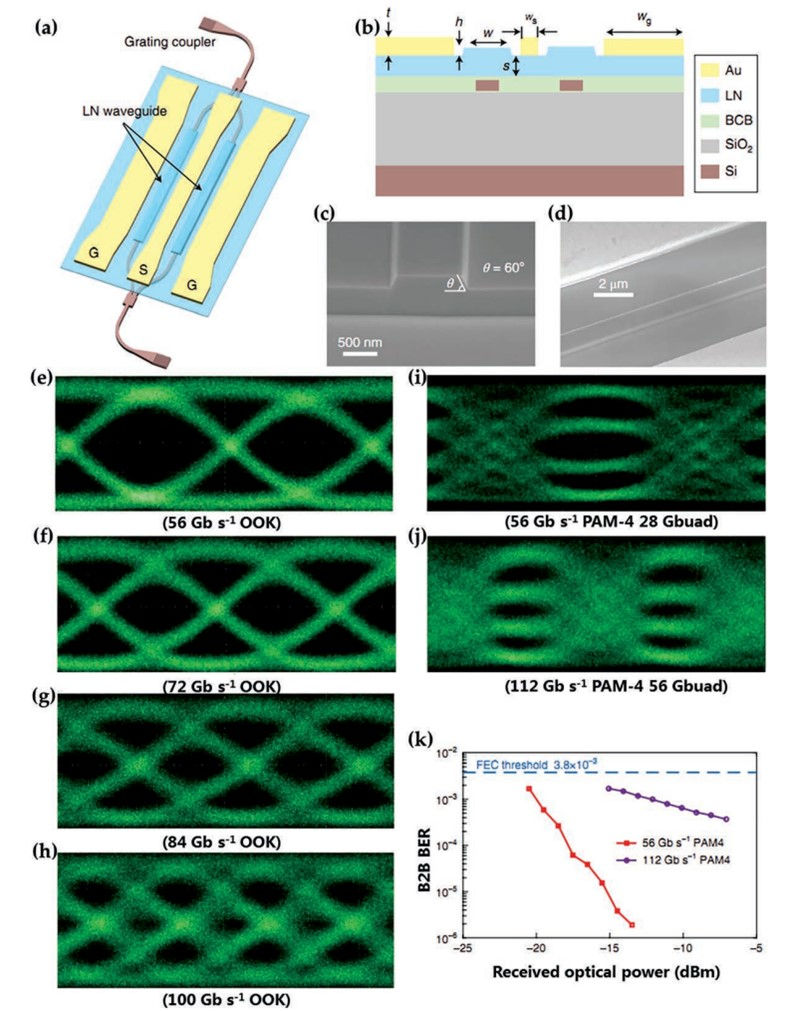
These results demonstrate that LNOI modulators can meet and exceed the demanding requirements of next-generation optical communication systems.
Electro-Optically Tunable Nonlinear Photonic Devices
The strong χ(2) and χ(3) nonlinearities of LN, combined with the high optical confinement of LNOI waveguides, enable a variety of nonlinear optical devices. Adding EO tunability to these components greatly enhances their functionality and reconfigurability.
Optical frequency combs are a prime example. Wang et al. demonstrated an integrated comb generator with an EO-tunable add-drop filter, as shown in Fig. 5:
The comb is generated in a dispersion-engineered microring, while the tunable filter allows precise selection and modulation of individual comb lines. This enables applications like flexible wavelength-division multiplexing for optical communications.
Fang et al. took this concept further by directly EO tuning the comb-generating resonator itself. Their device, shown in Fig. 6, allows continuous tuning of the entire comb spectrum:
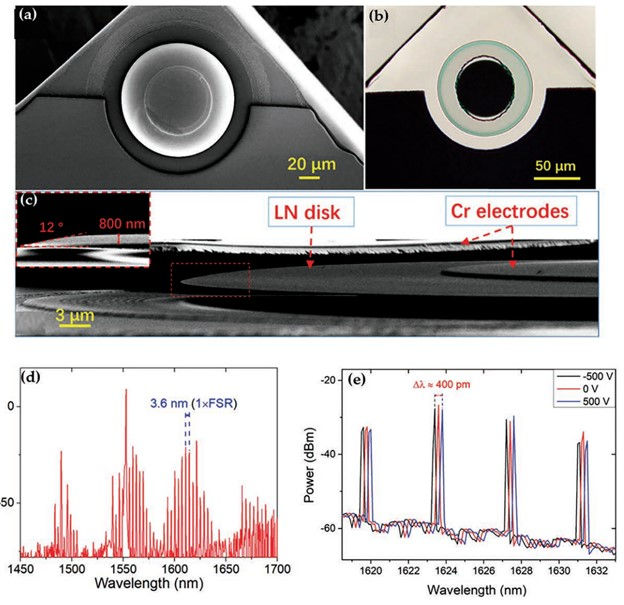
The electrodes integrated with the microdisk resonator enable tuning over a 400 pm range by varying the applied voltage from -500 V to +500 V. This opens up possibilities for adaptive and reconfigurable comb sources.
EO tuning can also be extended to control mechanical modes in optomechanical systems. Fang et al. demonstrated an LNOI optomechanical resonator with electrodes for tuning both the optical and mechanical resonances as shown in Fig. 7:

The device exhibits an optical Q factor of 10^7 and a mechanical Q factor of 2.86 x 10^8. The mechanical frequency can be tuned by -134 kHz per 100 V applied. This enables precise control of optomechanical interactions for applications in sensing and quantum information processing.
Photonic Crystal-based Devices
While most LNOI devices use ring or disk resonators, photonic crystal (PhC) structures offer even more compact footprints. Hu et al. demonstrated an EO modulator based on a PhC nanobeam cavity, as shown in Fig. 8:
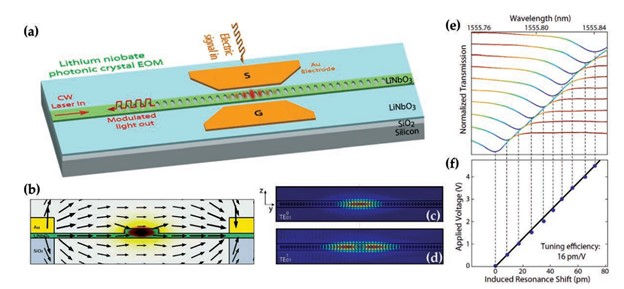
The device achieves a modulation bandwidth of 17.5 GHz with a tiny capacitance of only 22 fF, resulting in excellent energy efficiency. The compact size makes it ideal for large-scale photonic integrated circuits.
Design Considerations and Fabrication Techniques
Achieving high performance in LNOI EO devices requires careful optimization of multiple parameters:
Waveguide design: The cross-section and material stack must be engineered for low loss, appropriate dispersion, and good overlap between optical and RF fields.
Electrode configuration: Electrode placement and geometry should maximize the effective EO coefficient while minimizing optical loss and capacitance.
Material quality: High-quality LN thin films with minimal crystal defects are essential for maintaining strong EO coefficients.
Fabrication processes: Techniques like etching and metallization must be optimized to produce low-loss waveguides and high-performance electrodes.
Common fabrication approaches for LNOI devices include:
Photolithography and dry etching for waveguide definition
Electron-beam lithography for high-resolution patterning
Focused ion beam milling for fine-tuning of structures
Metal deposition and lift-off for electrode fabrication
Advanced techniques like chemomechanical polishing can produce ultra-smooth surfaces for ultra-high Q resonators.
Future Perspectives
While LNOI EO devices have made remarkable progress, there is still significant room for improvement and new applications:
Further optimization of waveguide and electrode designs to push the limits of modulation efficiency and speed.
Integration of active gain materials for on-chip lasers and amplifiers.
Exploration of novel device concepts like stationary-wave integrated Fourier transform spectrometers.
Development of large-scale, fully-integrated photonic systems leveraging the unique capabilities of LNOI.
Investigation of quantum photonic applications exploiting the strong χ(2) nonlinearity of LN.
Conclusion
Integrated EO devices on the LNOI platform have demonstrated remarkable performance, combining the strong EO effect of LN with the benefits of nanophotonic integration. From ultra-efficient microring modulators to reconfigurable frequency comb sources, these components are poised to play a crucial role in next-generation photonic systems. As fabrication techniques continue to mature and new device concepts emerge, LNOI technology promises to enable even more powerful and versatile integrated photonic circuits in the future.
Reference
[1] Y. Cheng, "Lithium Niobate Nanophotonics," Jenny Stanford Publishing, 2021.
コメント