Introduction
As computing demand continues to grow exponentially, driven by applications like artificial intelligence (AI) and 5G/6G wireless communications, existing electrical interconnect technologies are hitting fundamental limits. To overcome these bottlenecks, the semiconductor industry is turning to integrated photonics - the introduction of optical components directly into advanced CMOS processes. Combining optics and electronics onto a single silicon chip unlocks new capabilities and architectures that could enable the next wave of breakthroughs across computing, communications and sensing applications [1].
CMOS-Integrated Photonics
At the heart of these electronic-photonic systems is the ability to fabricate high-performance optical components like micro-ring resonators directly in a conventional CMOS process. Figure 1 shows an example of interleaved planar PN junctions forming resonant photonic micro-rings in a standard CMOS process flow. These highly compact (diameter ~10μm), high-Q (up to 200,000) structures can be used for a variety of functions like modulation, filtering, and sensing.
A key enabler has been innovations in advanced CMOS process technology itself. Figure 2 illustrates the evolution of a 45nm CMOS process to incorporate a variety of photonic features like germanium photodetectors, silicon partial etches, nitride layers for waveguides, and v-groove fiber couplers - resulting in a monolithic electronic-photonic platform operating at the same performance as advanced pure-play CMOS transistors.

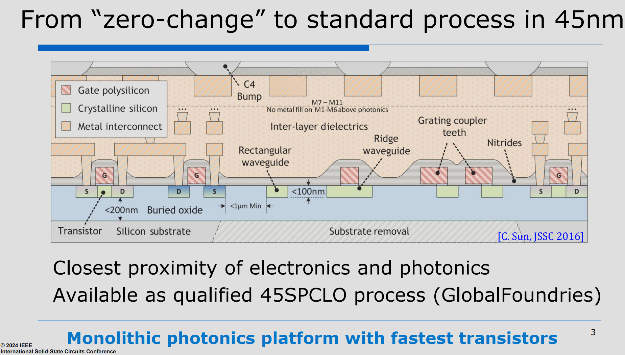
Applications and Impact
Digital Optical Interconnects for AI/ML Scale-out One of the most impactful applications of integrated photonics is in addressing the interconnect bottleneck for scaling out AI/ML workloads across large multi-chip/multi-package systems. As these models grow to trillions of parameters, the interconnect efficiency (ratio of compute time to total time including communications) drops from over 80% to below 60% when scaling out to multiple packages.
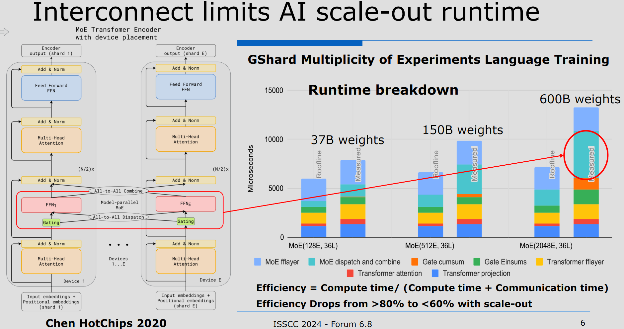
Integrated photonic chiplets like the TeraPHY from Ayar Labs (Figure 4) provide a revolutionary solution - a multi-wavelength optical I/O interface integrated directly into the package alongside the compute/memory chips. Each TeraPHY chiplet provides over 4Tbps of optical I/O bandwidth across 8 ports and up to 16 wavelengths per port (Figure 5). This dramatically improves system-level throughput and efficiency.
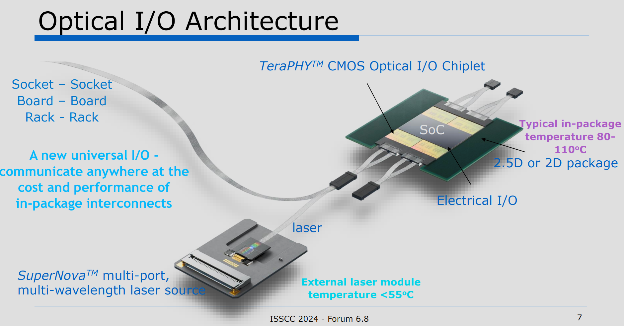
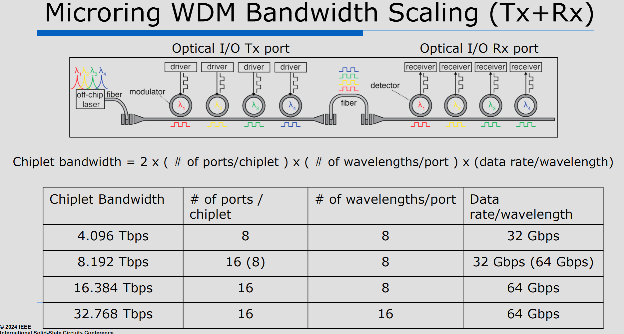
The TeraPHY chiplets leverage a disruptive new packaging approach (Figure 6) where high-bandwidth optical I/Os can be routed off-package through fibers and optical connectors. A SuperNova multi-wavelength laser source co-packaged with the compute chips provides the light source for communications within the rack, across racks, or to remote systems - enabling new distributed computing architectures.
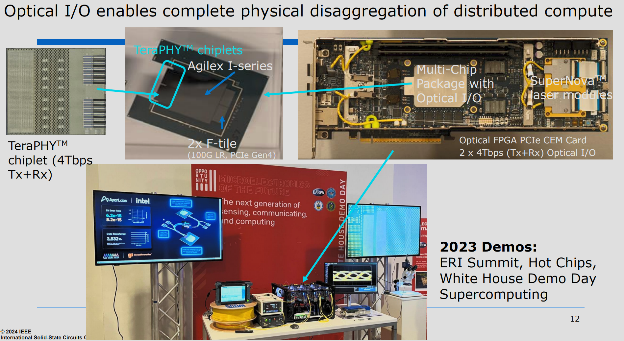
The technology roadmap (Figure 7) shows a clear path to reaching over 250Tbps of off-package optical I/O bandwidth within the next decade by leveraging advanced packaging technologies and scaled CMOS processes. This supports future AI fabric architectures like Nvidia's proposed system with 1 million nodes that are 100x faster than today's A100 systems (Figure 8).

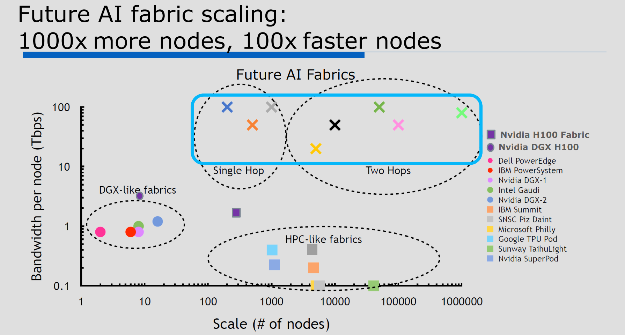
A key innovation enabling this scale is the SuperFabric optical interconnect architecture (Figures 9, 10) based on laser-forwarded coherent optical links. These links employ a novel coherent detection approach along with high-radix fast optical switches to provide over 100Tbps of connectivity to each package with fast reconfigurability.
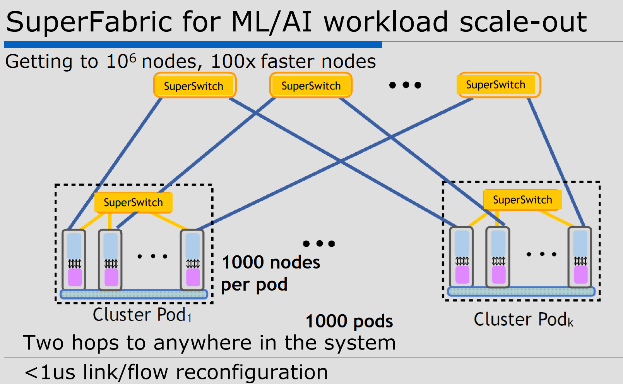
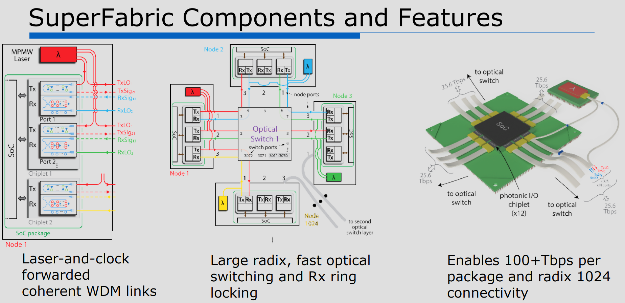
Analog/RF Optical Interconnects
Beyond digital computing, the convergence of photonics and electronics also enables transformative architectural approaches for applications like 6G/7G wireless and automotive radar sensing. The extremely high bandwidths and energy efficiencies of microring-based optical interconnects can be leveraged to disaggregate the analog/RF front-ends from the digital back-ends in systems like massive MIMO radios (Figures 11, 12).

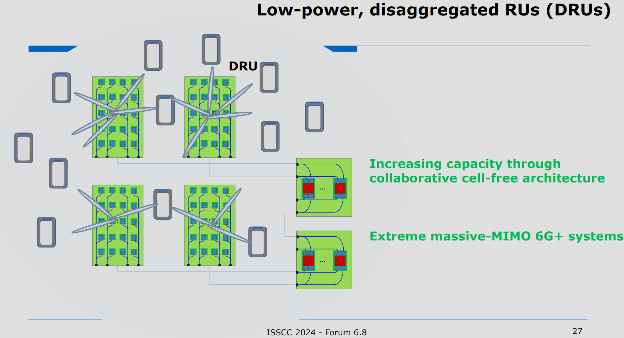
This architectural approach, combining digital optical interconnects to baseband processors with analog optical links to the distributed antenna/radar front-ends (Figure 13), dramatically reduces system power and footprint. Figures 14 and 15 show examples of implementing such analog optical links using high-performance microring modulators driven directly by millimeter-wave signals.
Figure 16 highlights the noise and linearity characteristics of single microring modulators as a function of laser power and wavelength detuning - identifying an optimal operating regime that can meet the stringent requirements of cellular and automotive radar applications. State-of-the-art prototypes have already demonstrated spurious-free dynamic range over 95dB.Hz2/3 (Figure 17), exceeding the 3GPP LTE requirement.
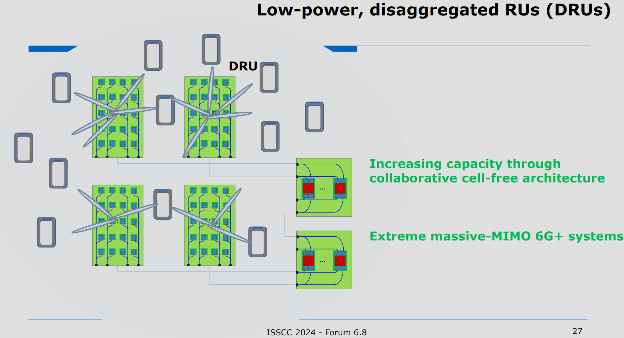
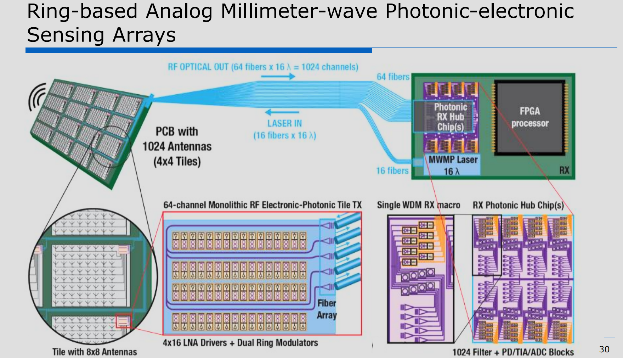



Cryogenic Interconnects and Sensing
Two other pioneering applications leverage the unique properties of integrated photonics in advanced CMOS processes. The first is an optical interconnect solution for cryogenic computing systems like quantum computers (Figure 18). Conventional electrical cabling quickly becomes impractical at scale due to thermal loads and wiring complexity.
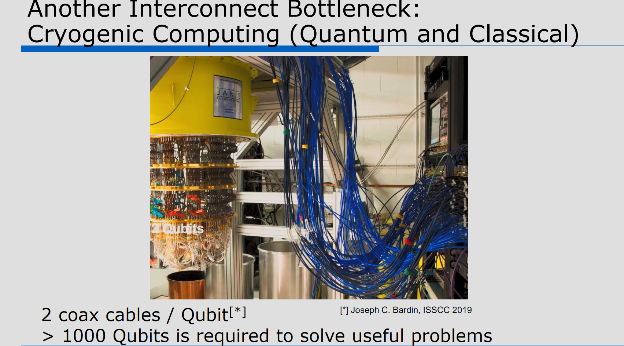
The Coherent Ultrafast Reflective Link (CURL) developed by researchers at UC Berkeley addresses this by using advanced CMOS electronics operating at cryogenic temperatures (Figures 19, 20) to drive ultra-compact microring modulators that reflect a multi-wavelength tunable laser back to a coherent receiver at room temperature (Figures 21, 22). First prototypes already demonstrate multi-Gbps link operation between 4K and 300K (Figures 23, 24).
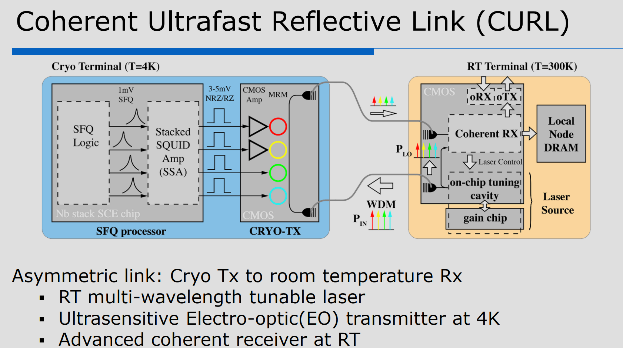
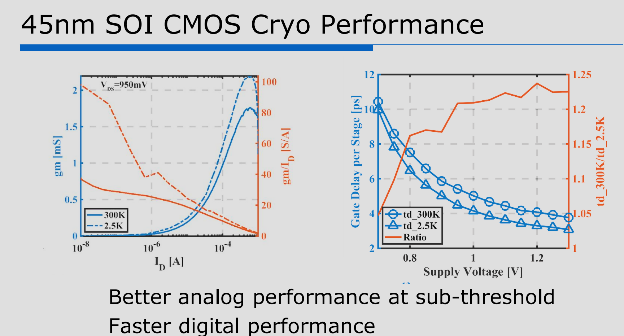
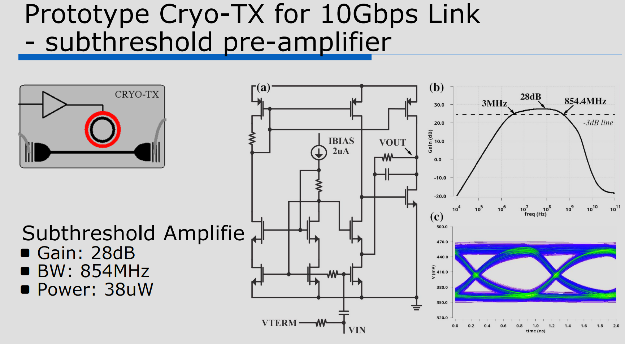



The second breakthrough application is in label-free photonic biosensing leveraging the high sensitivity of microring resonators to detect molecular and viral analytes (Figure 25). By monolithically integrating dense arrays of microrings with microfluidics and electronics (Figure 26), it enables highly multiplexed point-of-care sensing that could be extended into wearables and implantable devices for personalized healthcare applications.
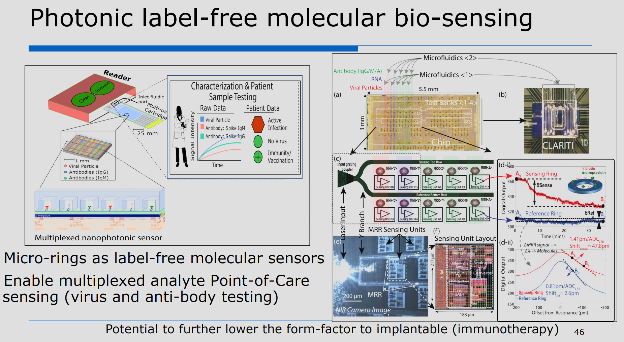
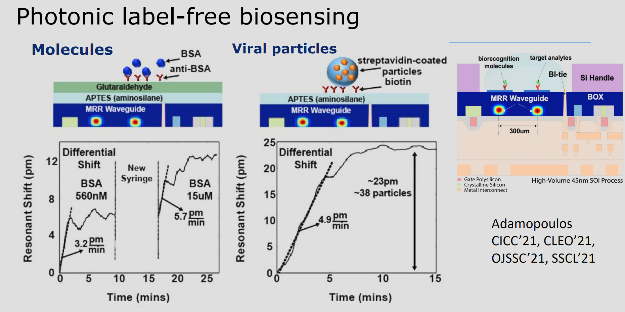
Conclusions
Integrated electronic-photonic systems are poised to revolutionize computing, communications and sensing by overcoming key bottlenecks with innovative new photonic device structures, link architectures, packaging and system-level solutions. While still in its early stages of commercial deployment, the field is progressing rapidly with clear roadmaps for scaling bandwidth density, energy efficiency and application scope over the coming decade.
Reference
[1] V. Stojanović, "Electronic-Photonic Systems-on-Chip for Compute, Communications and Sensing," in IEEE International Solid-State Circuits Conference (ISSCC), 2024.
Comments