Introduction
Quantum computers hold great promise for solving certain problems much faster than classical computers. However, building large-scale quantum computers capable of running commercially relevant algorithms remains a significant challenge. One approach to scaling quantum computers is through distributed architectures, where multiple quantum processor modules are networked together.
In this article, we will explore the use of silicon T centres as a platform for distributed quantum computing. T centres are optically active defects in silicon that combine excellent spin properties with the ability to emit photons in the telecom wavelength range. By integrating T centres into silicon photonic devices and connecting them via optical fibers, it becomes possible to create a scalable, modular quantum computing architecture.
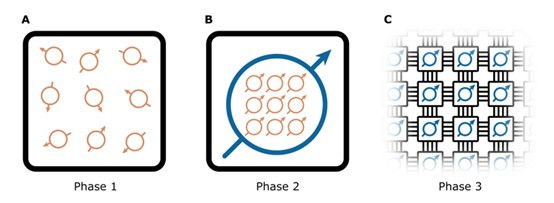
T Centre Basics
T centres consist of a hydrogen atom bonded to a carbon atom in the silicon lattice (Fig. 2A). The electronic structure of the T centre includes a ground state (GS) with electron and nuclear spin states, and an optically excited state (TX0) with a bound exciton. Under an external magnetic field, the optical transition splits into two spin-selective transitions (labeled B and C) that can be used for spin initialization, readout, and remote entanglement generation (Fig. 3A).
To create T centres suitable for quantum computing, isotopically purified silicon is used to eliminate unwanted nuclear spins. The T centres are then integrated into photonic cavities on silicon-on-insulator (SOI) chips, which enhances their optical emission properties via the Purcell effect (Fig. 3B-C).


Spin Control and Readout
Full control over the T centre spin states is achieved using microwave (MW) and radio-frequency (RF) pulses delivered via on-chip antennas. Electron spin transitions are driven using MW pulses (Fig. 4A-B), while nuclear spin transitions are driven using RF pulses (Fig. 4C-D). By applying appropriate pulse sequences, universal quantum gates such as controlled-NOT (CNOT) can be implemented.
Single-shot readout of the nuclear spin state is performed by mapping it onto the electron spin, which is then read out optically (Fig. 4E). This allows for high-fidelity state preparation and measurement (SPAM) of the nuclear spin qubit.
The T centres used in this work demonstrate exceptional spin coherence times (Fig. 5), with electron spin T2 times of up to 270 μs and nuclear spin T2 times of up to 220 ms. These long coherence times are crucial for implementing high-fidelity quantum operations.


Remote Entanglement
To enable distributed quantum computing, remote entanglement must be established between T centres on different chips. This is achieved using a protocol known as Barrett-Kok (BK) (Fig. 7A), which relies on the interference of photons emitted by the T centres.
The key requirement for successful entanglement generation is the indistinguishability of the emitted photons. This is quantified by the Hong-Ou-Mandel (HOM) visibility (Fig. 6), which measures the degree of quantum interference between photons from different T centres. By optimizing the spectral and temporal properties of the T centres, high-visibility HOM interference can be achieved.
Using the BK protocol, remote entanglement of T centre electron spins was demonstrated with fidelities up to 60% (Fig. 7B). The generated entanglement was then used to perform a teleported CNOT gate between the nuclear spin qubits of the remote T centres (Fig. 8-9), showcasing the potential for distributed quantum logic.
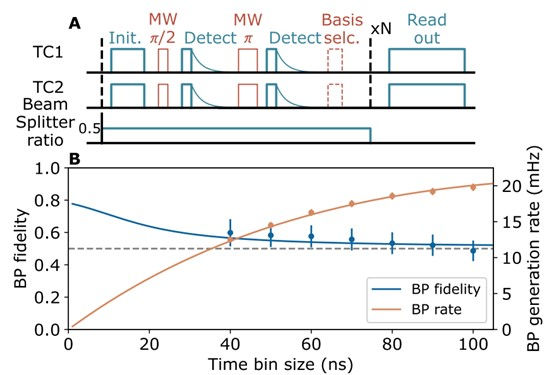
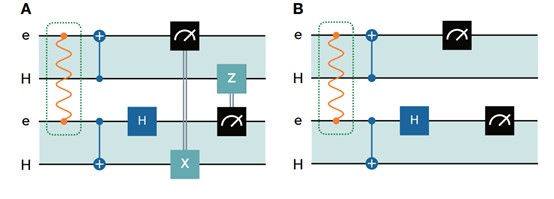
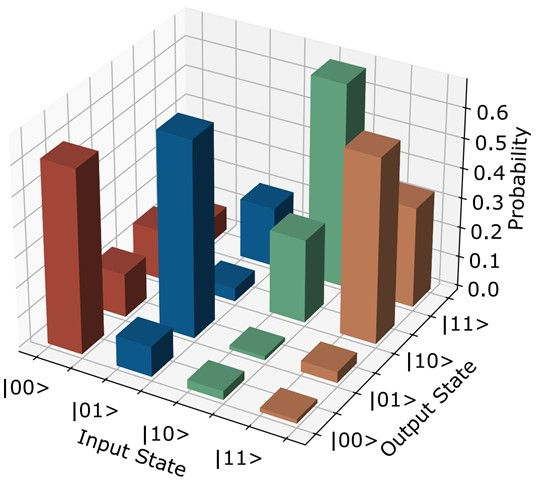
Outlook and Conclusion
Based on the experimental results and projected improvements in T centre devices, it is expected that remote entanglement fidelities of up to 99.9% and distribution rates of 200 kHz can be achieved (Fig. 10). This would enable fault-tolerant distributed quantum computing across multiple T centre processor modules.
Silicon T centres offer a promising platform for realizing distributed quantum computers. By leveraging the mature silicon photonics industry and the excellent properties of T centres, it may be possible to build modular, scalable quantum computing systems capable of tackling commercially relevant problems. The results presented in this tutorial represent important first steps towards this goal, paving the way for future advancements in distributed quantum computing technology.
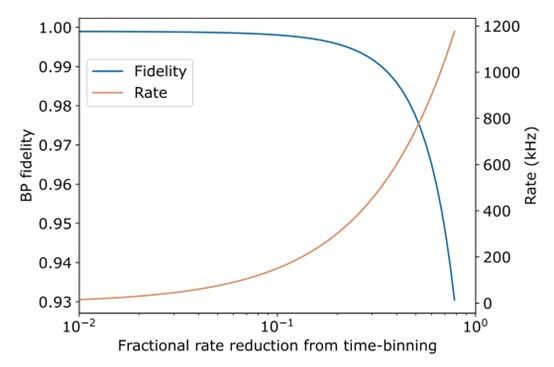
Reference
[1] Photonic Inc., "Distributed Quantum Computing in Silicon," June 11, 2024.
Comments